At noon every Tuesday from September through June, scenes from a revolution in neuroscience are playing out at Cold Spring Harbor Laboratory. Week after week, over 100 scientists cram themselves into a ground-floor meeting room in the Beckman Laboratory. It’s standing-room only as everyone in the Neuroscience Program settles in to hear details of the latest experiments being performed by their colleagues.
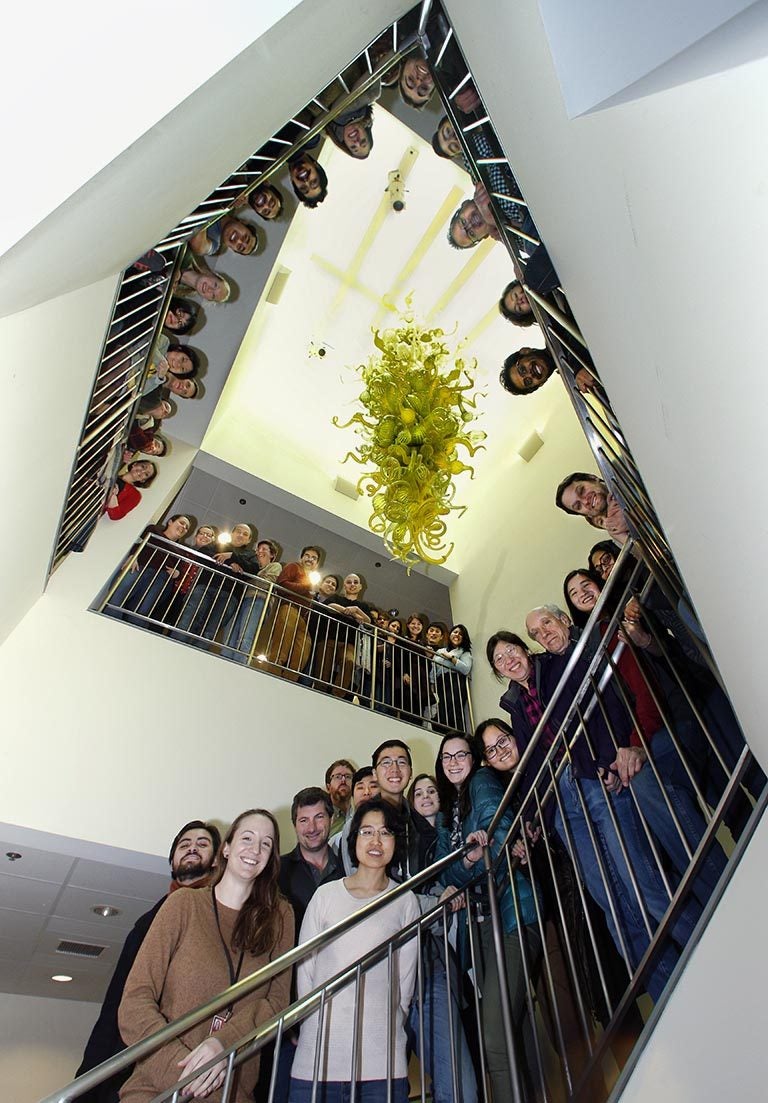
Over the course of an academic year, these “in-house seminars” provide the opportunity for every scientist in the group to know what every colleague is working on. They learn from one another, offer critiques, and riff on one another’s ideas. Casual suggestions can lead to collaborations and important innovations.
It’s all part of a historic effort to figure out how the brain works—that magnificent yet endlessly complex and therefore still mystifying structure that “is evolution’s greatest triumph,” in the words of veteran CSHL neuroscientist Josh Huang.
This past year, Florin Albeanu, whose lab studies how the brain processes olfactory information, and Pavel Osten, whose group is devising new ways of visualizing the brain, introduced to the rest of the faculty a new method called light-sheet microscopy. It was the fruit of a single comment Albeanu made after a presentation by a member of Osten’s team. “Ideas are cheap,” Albeanu says. “It’s easy to say, ‘I think you can do this another way.’ But making it happen means working together, in this case for years, to solve the many little problems that crop up in new technologies.”
This is how progress is made on the continuously advancing front of a scientific revolution. At Cold Spring Harbor, it’s the product of getting the right people together and giving them room to interact and innovate, with a minimum of constraints. “We’re an especially cohesive group,” observes Professor Anthony Zador, neuroscience program chair. “I always say that our group is greater than the sum of its parts.”
New technologies create new research opportunities.”
David L. Spector, Director of Research
The fact that a relatively small neuroscience group at CSHL is having an outsized impact on a dynamic and highly competitive field was recently reflected in a series of major grants awarded to faculty under the BRAIN Initiative managed by the National Institutes of Health.
Imagine being able to journey through the brain, from front to back. That’s exactly what light-sheet microscopy enables the Osten lab to do. This movie of the mouse brain was assembled by piecing together 54 thin linear “sheets,” imaged separately and later aligned and “stacked.” This will be invaluable in comparing brains to see how males differ from females, on average; how individuals of each sex vary; and how those with illness like schizophrenia differ from those who don’t.
CSHL President Bruce Stillman says the story is actually much bigger, as the Laboratory is now seeing major returns on investments in neuroscience that have been made over a decade’s time. Each has involved committing substantial resources to individual investigators, based on novel, high-risk, high-reward ideas they’ve advanced. The approach is therefore “classic Cold Spring Harbor,” bringing to mind the first days of the Laboratory’s modern cancer research program in the late 1960s and its initial foray into neuroscience during the late ‘70s and early ‘80s.
Big bet on rodents
“About a dozen years ago we consciously re-invented ourselves,” says Zador, “focusing on a high-risk research program that we referred to as ‘rodent cognition.’ The aim was to understand the neural mechanisms by which animals perform complex behaviors, using rodents as a model system.” While most of the global neuroscience community has by now seen the light, only a few years ago “there was lots of skepticism about whether it would work,” Zador says.
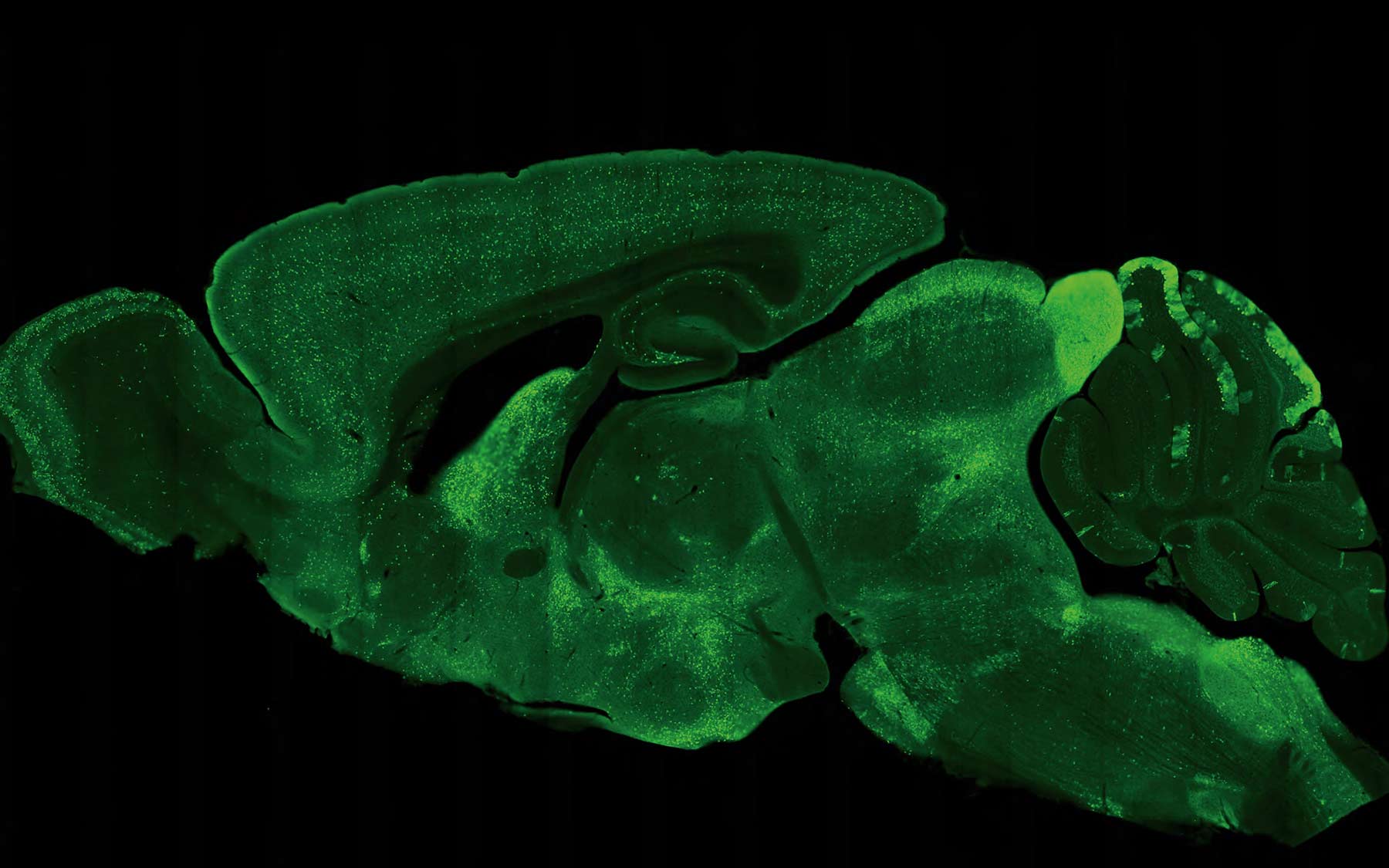
The idea was to use rodents to learn about higher brain functions that many believed could only be studied in monkeys and chimps. Zador and others at the Laboratory had a problem with that. A typical primate experiment was performed on only two subjects at a time and took years to complete. Rodents, in contrast, were easy to breed, readily available, and easy to work with.
What made the decision to focus on rodents seem inspired was the emergence of an array of tools and technologies with which to probe the rodent brain—which despite its comparatively small size is remarkably like the human brain, sharing key features of anatomy and genetics.
In 2005, researchers at Stanford demonstrated optogenetics, a technology that would take the neuroscience world by storm. It enabled experimenters to use beams of colored laser light, delivered painlessly through hairlike optical fibers into the brains of living rodents, to selectively switch individual neurons on and off.
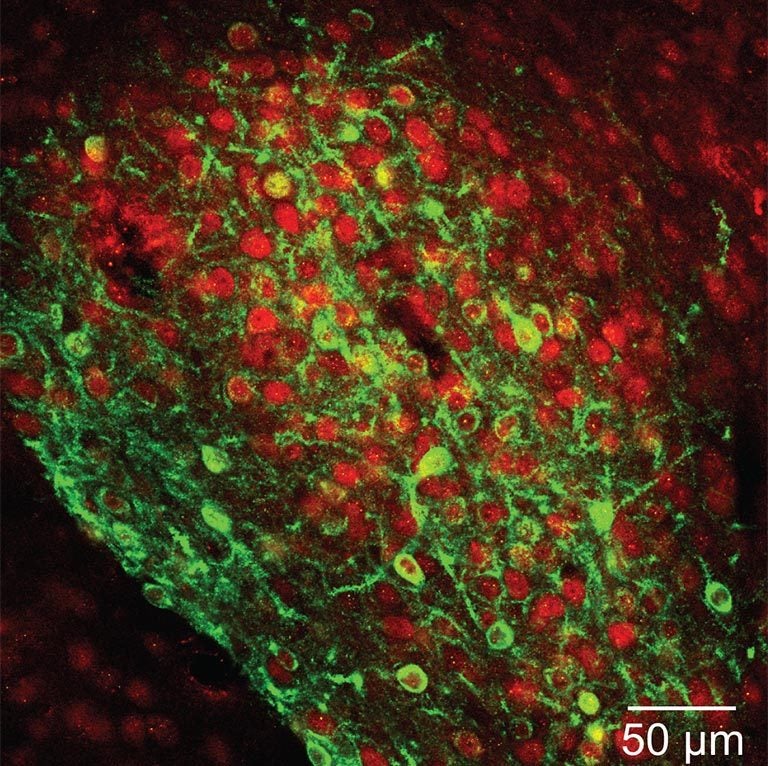
Bo Li is among the CSHL neuroscientists who have used optogenetics, along with other tools, to make important discoveries about how specific brain areas and cell types are implicated in specific behaviors. In tracking down the precise area of the brain that governs fear learning and memory—the central amygdala, it turns out—the ability to optically probe genetically defined groups of neurons was vital in Li’s work because there are two sets of neurons important in fear-learning and memory processes. The difference between them, his team learned, was in their release of message-carrying neurotransmitters into the spaces called synapses between neurons. In one subset of neurons, neurotransmitter release was enhanced; in another it was diminished. If measurements had been taken using older methods across the total cell population in the central amygdala, neurotransmitter levels from these two distinct sets of neurons would have been averaged out, and thus would not have been detected.
Not only could brain cells be controlled with light; chemogenetics made it possible to control individual neurons with engineered receptors and molecular activators. At CSHL, a genetic method called cre-lox recombination also had a great impact. It enabled Josh Huang to engineer two dozen mouse lines that each expressed fluorescent “reporter” molecules specific to individual cell types in the brain. One could image the entire brain and see every cell of a certain neuronal species glowing with green fluorescence. Other tools included deactivated viruses used to trace the sinuous, branching pathways that these cells deploy to communicate with one another.
“All of these methods made the mouse, in particular, the preeminent system for understanding neural circuits,” says Zador. “We became equipped with techniques and tools to dissect an animal’s behavior at a level we couldn’t have imagined before 2005.”
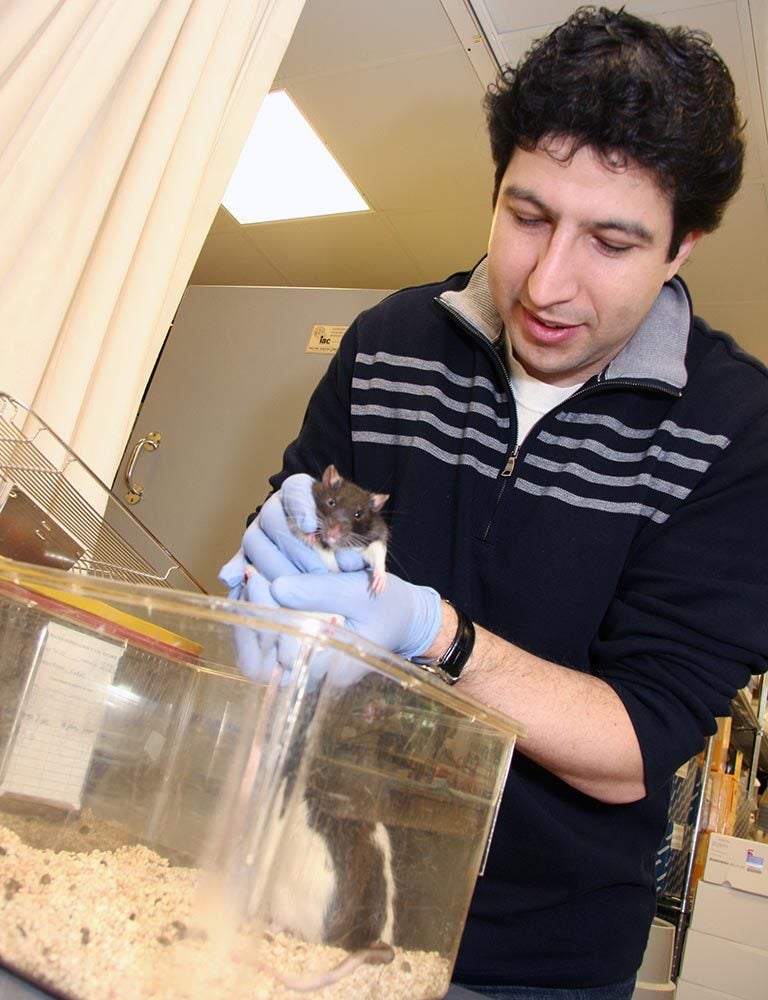
A vivid example is the research of Adam Kepecs and team. They have used rodents to study confidence, a brain-based phenomenon so difficult to grasp that we usually refer to it as a “feeling.” How do we know when a rat is exhibiting confidence? Kepecs and colleagues devised a method to study decision making in these animals. The rats were offered an odor that they were trained to associate with one of two doors. When they chose the correct door, they were rewarded. This part was easy for the animals: their selections were almost always correct. Things got trickier when the rats were offered a mixture of the two scents, with one dominating over the other by only a very small percentage. The rats now needed to choose the door representing the dominant odor in order to get their reward—a choice that reflects their best guess.
The innovation in this work was that Kepecs was able to measure confidence simply by challenging a rat to wait for the reward to be revealed behind the door. The time they were willing to wait served as a measure of their confidence in their original decision. “This is something that we can measure and create mathematical models to explain,” he says. And indeed he has, in subsequent work. It reveals that rodent and human brains are constantly processing data to make statistical assessments that translate into the feeling we call confidence. It’s a required first step to figure out how nerve cells in specific brain regions actually perform this operation.
The team that has made reality of the “rodent cognition” concept (and much more) was put together by CSHL President Bruce Stillman. Over the last decade, new faculty, bearing new ideas, have been brought on board. A great deal of research has been published. Stillman, in describing the state of neuroscience at the lab right now, acknowledges his predecessor. “Jim Watson was right on the cusp,” he says. “It seemed as if research had come to a fork in the road. Jim realized that there was no fork. The only way to move forward in biology was to take the molecular path. That’s what happened in cancer research. But it also is the key fact in understanding how our research on the brain has advanced so remarkably.”
Director of Research David L. Spector explains that the application of molecular biology techniques to the brain has given us a handle, literally, on dissecting its thicket of cells, circuits and networks. “Understanding the brain means understanding both the parts and the whole that they comprise,” he says. “It’s like a puzzle—taking the pieces apart, which many of our scientists have done; and putting them back together, which others focus on, to see how they work together as an ensemble that gives rise to consciousness, self-awareness, and thought.”
CSHL’s edge
“Science itself is always evolving,” Spector points out, “in part because new technologies create new research opportunities. We have transitioned into new areas that have since exploded with activity.”
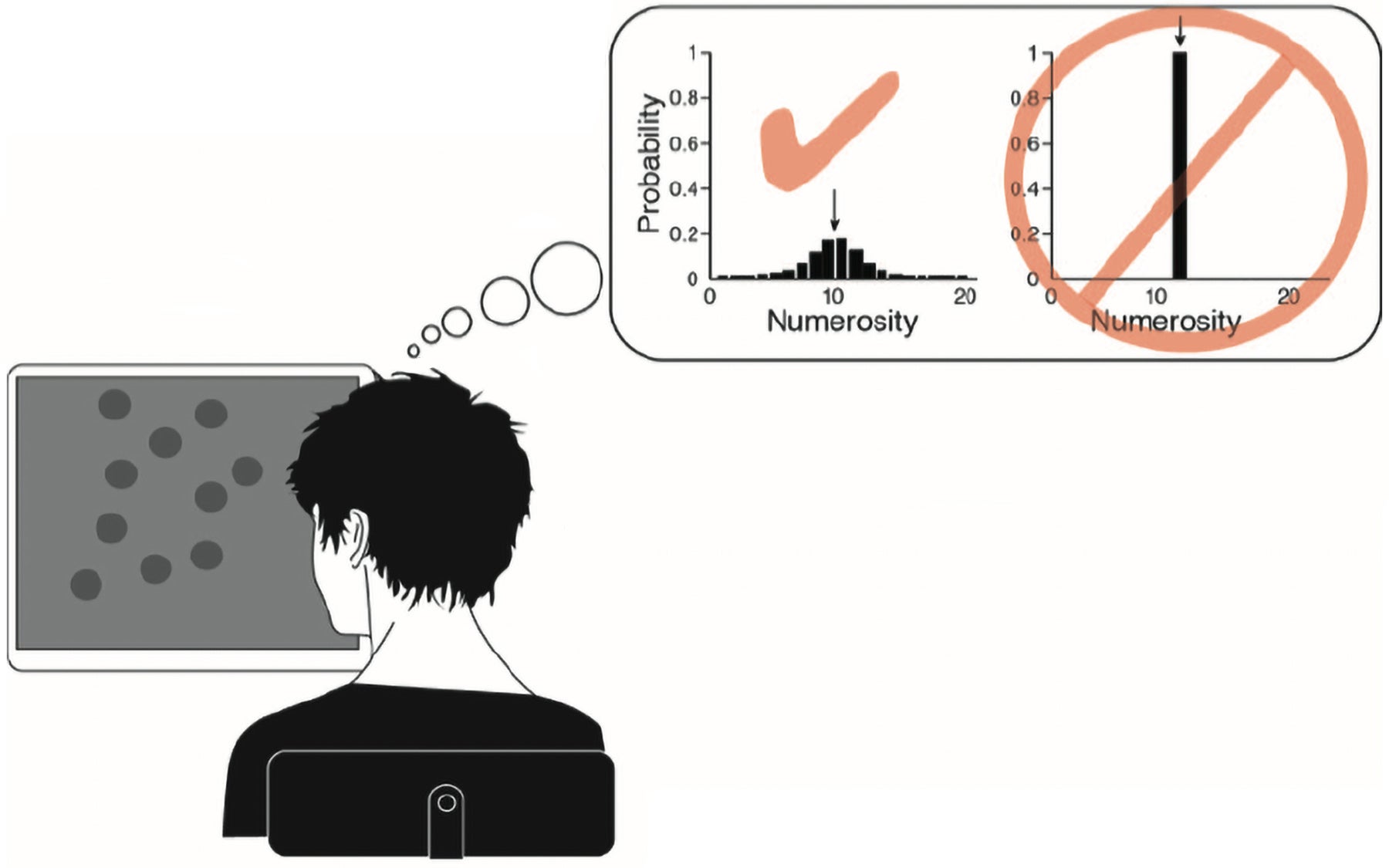
CSHL neuroscientists have pioneered the use of several of the new technologies, and were early adopters of most of them, according to Tony Zador. “So now the kinds of things that we’ve been doing for 10 years are just becoming standard at many other places.” This has been a great aid to recruiting the best young scientists, as “they see that the action is in the kinds of stuff that we’re doing,” Zador says.
This helps explain how the Laboratory attracts talents like Anne Churchland, whose postdoctoral work at the University of Washington was in primate cognition. Churchland has devoted her lab at CSHL to studying decision-making in both rodents and people. Her innovative approach to figuring out how the brain processes information flooding in from multiple senses is now part of the work of the International Brain Laboratory or IBL, of which the Churchland lab is one of 21 founding members. They’re trying to understand how the brain to combines a vast array of information from prior experience, current sensory stimuli, and internal and environmental contexts, using computational tools to help solve this massive problem.
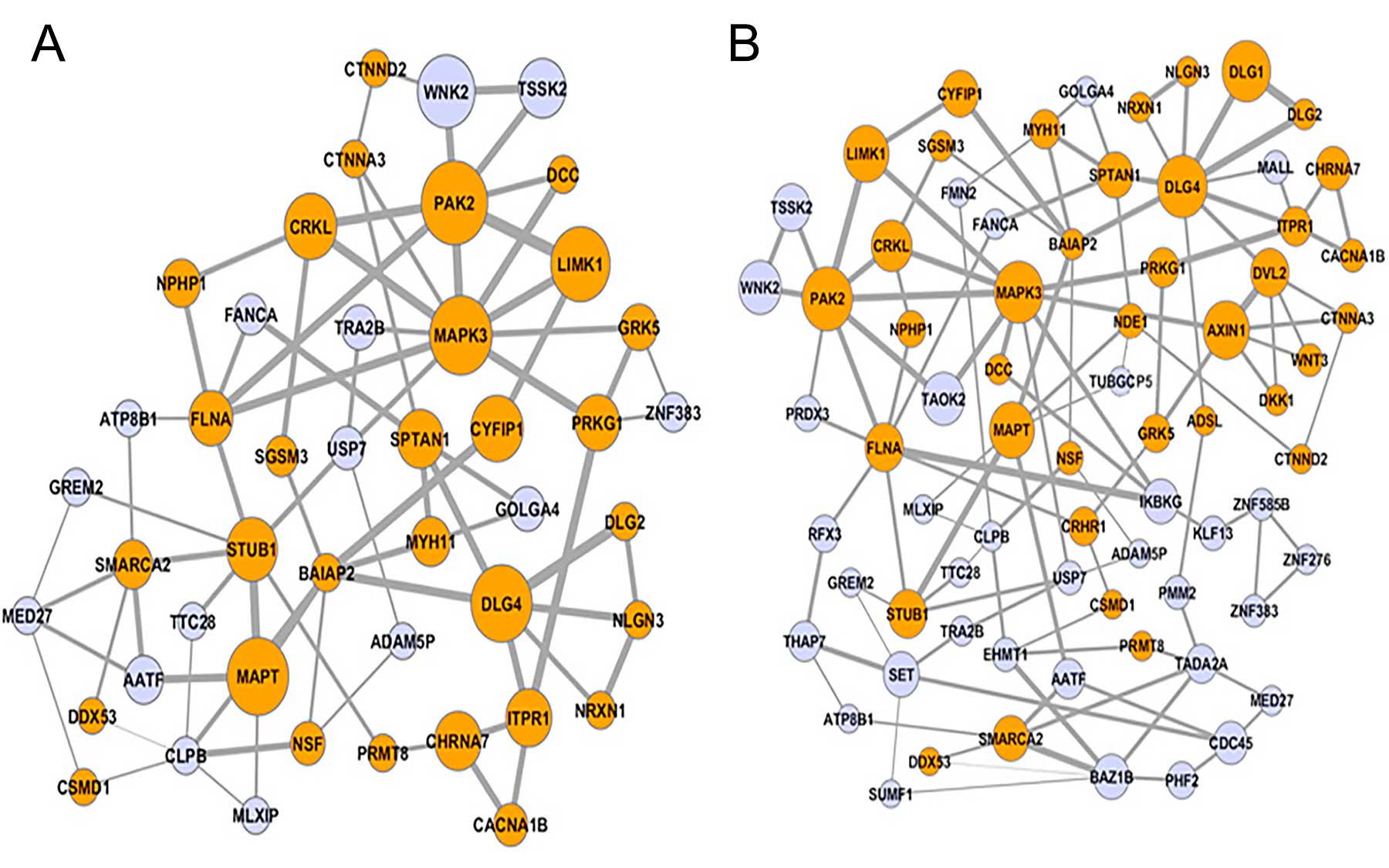
Computational biology, in which the Laboratory has made a significant investment in the last decade through the work of the Simons Center for Quantitative Biology, informs the work of the neuroscientists at a deep level. Two members of the neuroscience faculty, Alexei Koulakov and Tatiana Engel, work with data generated by experimentalists to theorize about how the brain is able to do what it does, and how the architecture of its circuitry is related to its astonishing functional capabilities.

Ivan Iossifov, Jesse Gillis, Dan Levy and Michael Wigler, all of them gifted in computational analysis, play key roles in research aiming to uncover the genetic underpinnings of illnesses from autism to schizophrenia and bipolar disorder. Dick McCombie, the pioneer gene sequencer, has led his team in multiyear studies of specific “risk” genes in bipolar disorder and schizophrenia.
Apart from technology, the human factor is also critical. Years of experience at CSHL have convinced Director of Research Spector that “it is probably the most interactive scientific environment in the world,” a fact he attributes to the lack of formal academic departments. “It’s one big group, and the keynote is interacting and taking advantage of exciting discoveries and approaches that your colleagues are using and bringing them into another field.”
His favorite example—enthusiastically seconded by President Stillman—is what might be called the “molecular biologization” of Zador’s research. An expert on the auditory cortex in the mouse brain, Zador some years ago was influenced by informal discussions with CSHL colleagues about the use of genetic and viral technologies in cancer research. This was the spark that ignited five years of research in his lab, culminating a year ago in their introduction of a strikingly original way of mapping brain circuits. Called MAPseq, it converts the thorny problem of tracing the paths of individual neuronal projections—the axons that carry their information to other cells in the brain—from an optical problem into one of DNA sequencing. On the horizon is BARseq, a related method for mapping the projections of thousands of spatially resolved neurons by combining the high throughput of DNA sequencing with the high spatial resolution of microscopy.
“This is a fabulous innovation,” says Stillman, “that is the result of Tony getting good input from molecular biologists on our faculty about creating identification tags that we call genetic barcodes, and figuring out how to express them in individual neurons.” Stillman adds that finding new ways to map the brain were a subject of interest at the Laboratory years before a wave of “connectome” projects swept the neuroscience world. He cites an influential 2008 meeting at Banbury Center out of which emerged a proposal by Partha Mitra. To understand how the brain works (or fails to work in disease), it is “critical that we understand its wiring diagram more fully,” Mitra said in a white paper published the following year, a road map for his Mouse Brain Architecture project.
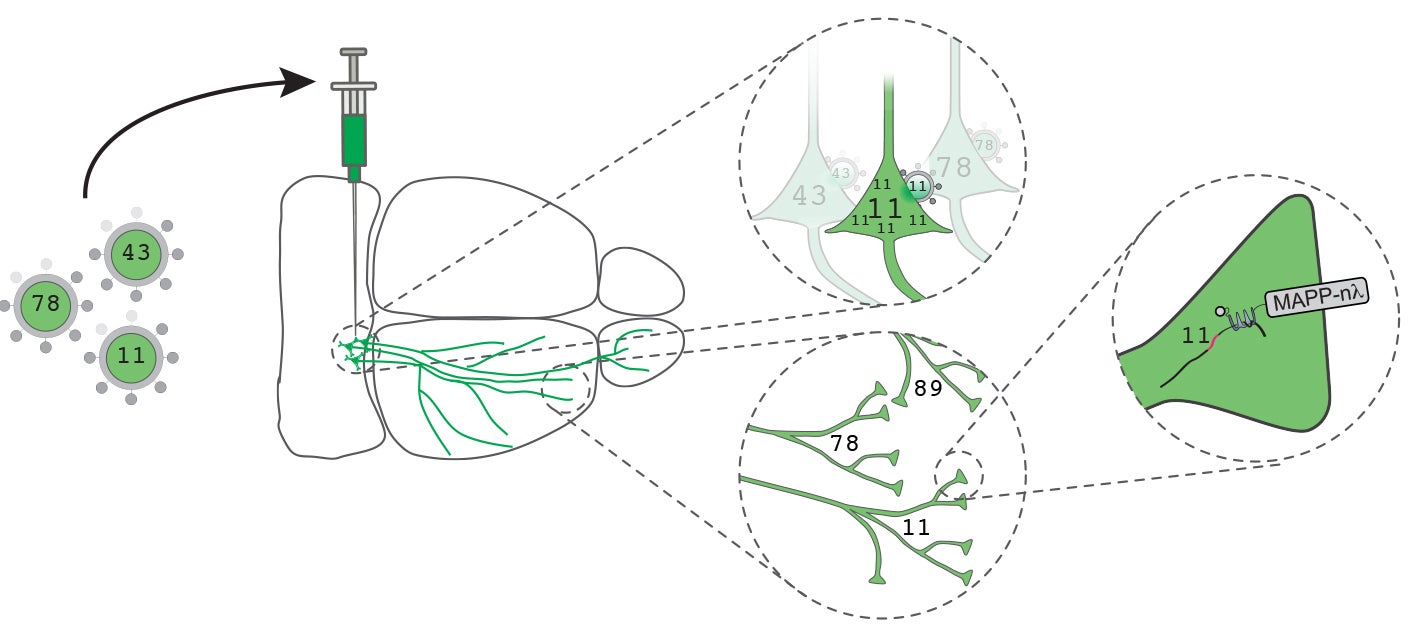
Zador, for his part, wanted to see if brain circuits could be mapped much more rapidly and cheaply than available technology allowed. With MAPseq’s barcoding technology, a single experiment using a single mouse and requiring only a week of work and several tens of thousands of dollars can reveal the destinations of hundreds, thousands, even tens of thousands of neurons. This next-gen way of mapping the brain reveals for every barcoded neuron the location of every part of the brain it is communicating with. It’s a fine example, notes Spector, of how new technologies bring new kinds of information to light.
Zador’s rapid and economical MAPseq method makes it feasible to compare differences in the brains of different individuals, individuals of different genders, and individuals (mice in this case) that model human illnesses. The team has such mouse models to work with as a result of other faculty collaborations. CSHL geneticist Alea Mills has bred a line of mice that carries a powerful genetic mutation strongly implicated in a subset of people with autism. Beyond studying the behavioral impacts of the mutation, it is possible now to use various brain mapping methods to see how such mutations change brain biology—providing, it is hoped, targets for future therapies.
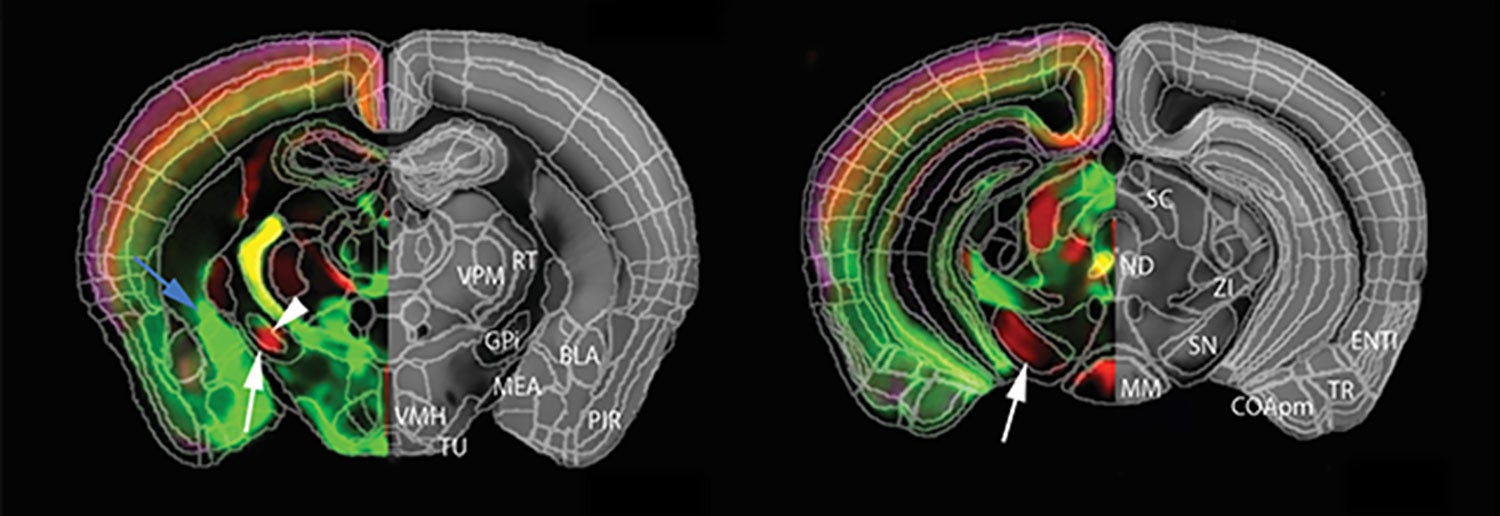
Such mapping experiments are in progress in both Zador’s lab and in the lab of Pavel Osten, who has also developed a novel method of looking at the brain called serial two-photon (STP) tomography. It too has caught on in labs across the world. By automating and standardizing the process in which brain samples are divided into sections and then imaged sequentially at precise spatial orientations in two-photon microscopes, Osten has succeeded in making whole-brain mapping mostly robotic, and therefore routine.
By tweaking STP, Osten’s team has made it possible for the first time to accurately count how many neurons are active in various brain areas during behavior. Another elaboration of STP enables Osten to comprehensively count the total populations of specific types of cells throughout the mouse brain. The first of these experiments yielded unexpected information about how the brain differs in males and females.
Sex-specific differences in the brain emerge during development, a focus of research by Jessica Tollhukn and colleagues. They join veteran CSHL experts on brain development, Josh Huang and Linda Van Aelst. Huang has shown how various subtypes of brain cells migrate to places in the cortex at key moments early in life. Van Aelst, an authority on how neural cells are generated by precursors and how they use specialized proteins to communicate, has shed light on how aberrations in signaling involving enzymes called small GTPases can result in diseases, including mental retardation.
Tollkuhn’s research has demonstrated for the first time the specific hormone receptors, brain cells and brain regions responsible for masculinization in the mouse. It’s part of her lab’s larger project aimed at understanding how hormones define distinct neurodevelopmental trajectories in male and female brains.
Doing the near-impossible
In another example of improbable collaboration at Cold Spring Harbor leading to important discoveries, teams led by neuroscientist Stephen Shea and biochemist Nicholas Tonks began several years ago to work on different aspects of a common problem—the devastating illness Rett syndrome, an autism spectrum disorder. It all began when a postdoc in each lab had an informal chat at lunch. The neuroscientist explained a difficulty in her research to the biochemist; the latter related the problem to one that he had dealt with in a different context. This launched separate projects on Rett that led to two publications. One, from Shea’s lab, demonstrated in mouse model of a Rett how failure of a gene called Mecp2 has biological consequences that impair learning in adult females. The other, from Tonks’ group and also based on mouse experiments, showed that candidate compounds could ameliorate several behavioral symptoms of the disorder in female mice.
“The question is: would this kind of cross-fertilization happened somewhere else? I’m not sure it would have,” says David Spector. “It’s an example of what makes Cold Spring Harbor such a unique place to do science.”
“I call it the CSHL way of doing things,” Tony Zador says. “It’s something about the atmosphere, and a reason we’ve been at the cutting edge for so long. We complement each other. We don’t always work on the same projects, but we influence one another. New technologies get shared, quickly. The overall intellectual atmosphere makes possible here what’s near-impossible elsewhere.”
Written by: Peter Tarr, Senior Science Writer | publicaffairs@cshl.edu | 516-367-8455