Comprised of a M.C. Escher-esque network of pathways, the brain is a maze of chaotic proportions. In its full-functioning glory, the brain helps us move, think, dream, and create. It’s a beautiful marvel of evolution that coordinates and translates messages from cells into emotions, memories, and actions.
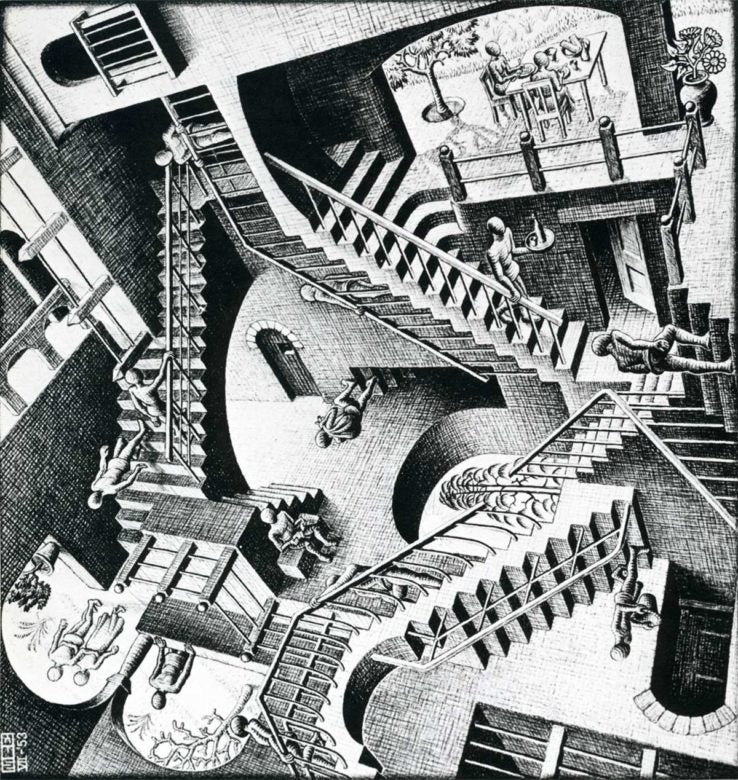
Li first started on his odyssey into the abnormal brain as a medical student in the 1990s. Originally intending to train as a clinician, his trajectory shifted after clinical rotations in a psychiatric hospital. The sheer helplessness felt by both the doctors and patients, as well as the lack of technology to probe at the problem presented Li with an obvious knowledge gap.
“It was striking to me that they had maybe three drugs as options for treating these patients, and they didn’t know if any of them would work,” Li said. “But what could they do? The only measurement the doctor had was behavior observation, and by talking to the patients. It made me think: how can we try to understand, how can we change that?”
The confluence of curiosity and frustration set him down a path into neuroscience research.
It’s a good time to be doing neuroscience research on mental illness, commented Li, smiling, his eyes hopeful. He thinks this is a lucky era for the field, a golden period in scientific time where a lot of technology, innovation, and ideas are converging. And even though ideas like using MDMA to treat PTSD are a bit out-there, he thinks it’s good there’s more attention being paid to finding effective ways to treat mental illness.
Just a month ago, the FDA approved a ketamine-derivative nasal spray as a therapy for depression. Its presence breaks a more than 30-year-long dry spell from when the last drug for depression entered the market. “Most of the therapies on the market are not based on our knowledge of how the brain works, or the mechanism of the disease. Most drugs are based on serendipity,” said Li. “The drug was used for some purpose first and then people eventually discovered that maybe it’s important for depression. A good example is ketamine. Ketamine is a pretty hot drug right now. But it was not initially used for treating depression, it was used for anesthesia, and someone discovered that people waking up from anesthesia are sometimes relieved of depression symptoms.”
Additionally, according to Li, many of these clinically available drugs are not perfect. They’re not effective for many patients, and can have unappealing side effects. To overcome these, Li said that we have to go back to the basics, and work to tackle the biological roots of these diseases, like the brain circuits that he and many other neuroscientists have zeroed-in on. Better understanding of the circuits involved in disease could mean better drug targets. That’s what he hopes is in store for the next-generation drugs for mental disorders.
Although at times precarious, exploring the fringes of psychological treatment does yield some positive effects, Li admits. “Those drugs, although not discovered by knowledge based on understanding of the brain, actually provide a lot of clues about what might be involved in the disease,” Li said. Dopamine from opioids, and serotonin from MDMA are good examples, he listed. Ketamine and the NMDA-receptor interaction is another. “On the one hand, it gives us avenues, ideas, hypotheses to test. On the other hand, it can limit our thinking, so we need to be open to new biological possibilities of what has been changed in a disordered brain that’s not just on the periphery,” said Li.
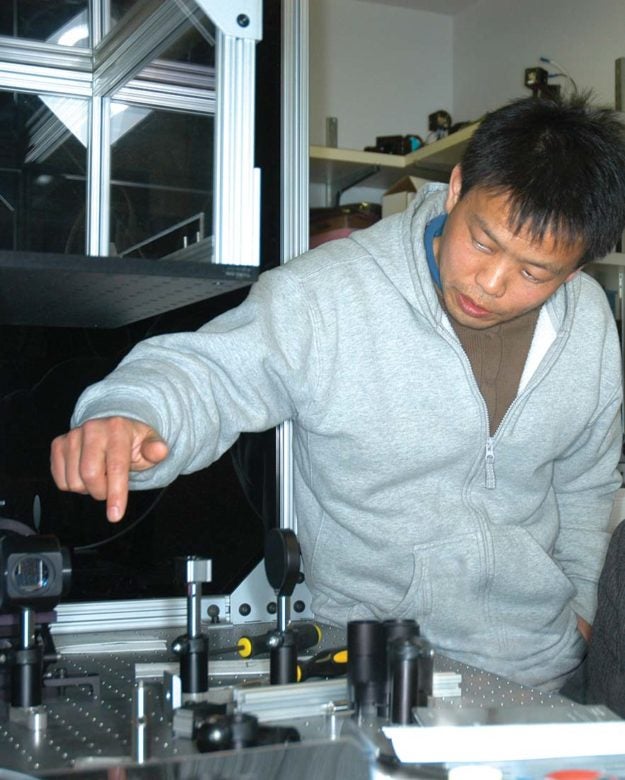
Now a professor at Cold Spring Harbor Laboratory, Li first arrived in 2003 as a postdoctoral fellow in Professor Roberto Malinow’s lab. Li’s research has always been linked to disease, influenced by his clinical background. His interest is studying behaviors most affected by human diseases like motivation, emotion, learning, and memory. He wanted to understand how a particular brain circuit (the lateral habenula circuit) controls emotion, motivation, and how dysfunction in the circuit may lead to mood disorders or disorders of motivation, such as depression (pdf).
He thinks neuroscientists could not even begin to scratch the surface of these kinds of questions without the advent of groundbreaking innovations that have altered the landscape and the nature of neuroscience research.
“I think two key technology developments play a very important role in our research. One is genetics. Mouse genetics and human genetics. The mouse genetics technology allows us to target a brain structure or even a cell type in that brain structure, very precisely. You can not only see this neuron in the brain, how they are active in different situations, but also, control those neurons’ activity, and change the activity of this neuron to see how it contributes to behavior.” Li explained. “In disease situations, we can reverse those changes, and see if behavior can be normalized.”
To instigate these genetic changes, Li and other neuroscientists rely on optogenetics and chemo-genetics. “With optogenetics, you can use a light to precisely control the activity of a neuron–excite it, inhibit it–in real-time. Chemo-genetics uses chemicals to treat the animal so that to affect the neuron activity in a less invasive way. The combination of these two technologies really enabled us to understand how the brain circuit controls behavior,” he said.
On the origin of fear: Your brain on anxiety
Since starting his own lab in September 2008, Li’s interests branched in two directions: one is to continue the work on the circuit that controls motivation and depression and the other one is on another circuit that has been long hypothesized to be important for fear-learning. The dysfunction of the latter is thought to be important for anxiety disorders. That circuit is based in a core structure in the brain, called the amygdala.
Heart beating fast; short, staccato breaths: classic signs of the body revving up the sympathetic nervous system’s fight-or-flight response. It’s an evolutionary induction of anxiety that has proven in the past to be a vital defense mechanism. However, the presence of anxiety when there are no threats is indicative of maladaptive anxiety behavior, crossing the threshold into pathological anxiety, as exhibited notably in patients with post-traumatic stress disorder, or PTSD.
Let’s backup to the 1930s. The first clue that the amygdala was important for fear-learning came from the observation of uniquely fearless monkeys. Scientists found that monkeys with lesions in their amygdala were unafraid of threats from snakes and other natural predators. It led scientists on a hunt to understand why the amygdala can control critical emotions like fear. A lot of this relied on designing mouse-sized anxiety tests proctored in a controlled laboratory environment, and subsequently monitoring the biological and behavioral outcomes.
If you give a mouse an anxiety test, you can see how fear is made and unmade.
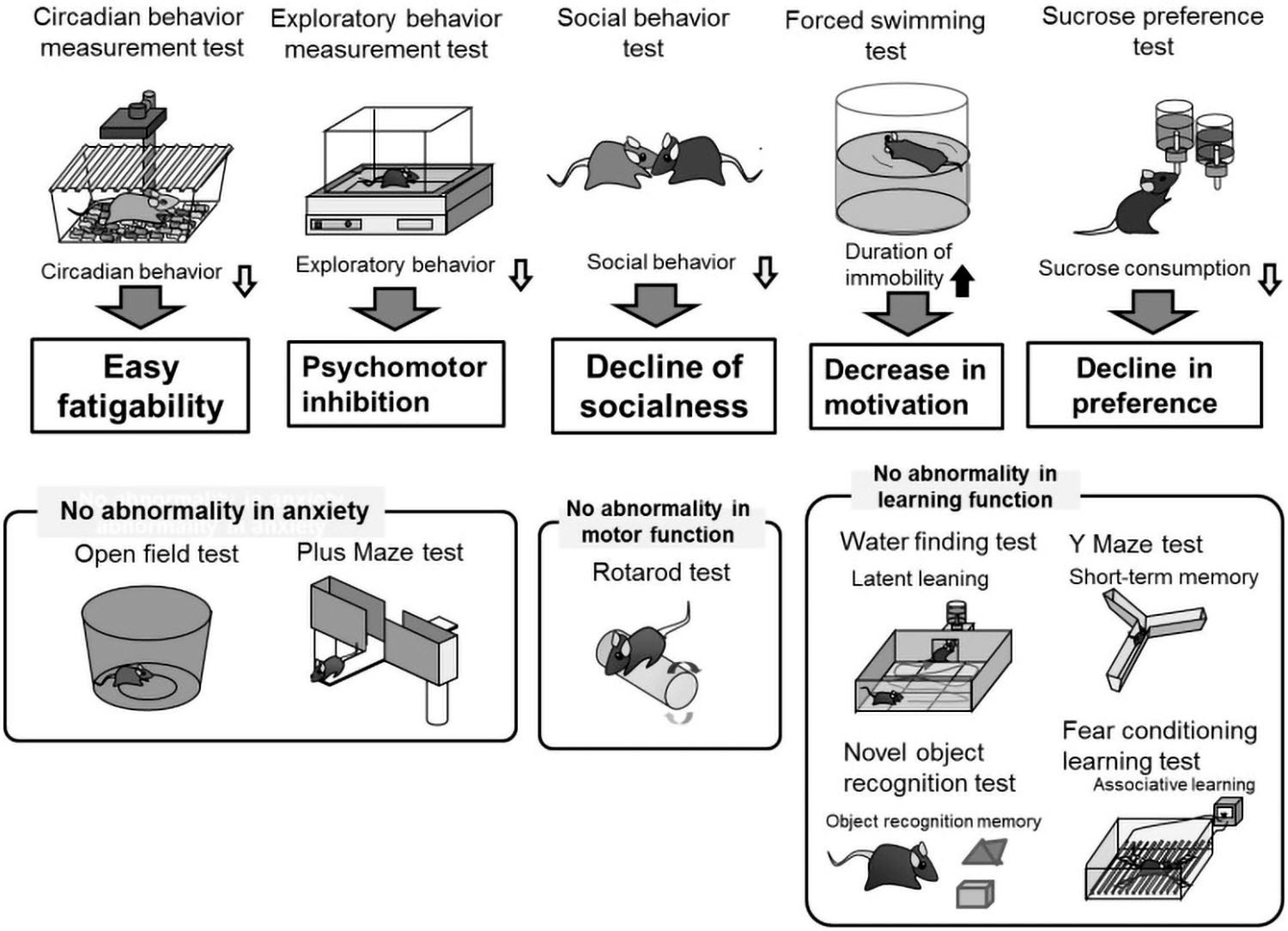
“People use simple behavioral paradigm such as fear conditioning: basically using a sound paired with a foot shock, to train a mouse in the lab. Then, when they hear the sound, they become afraid of it. So this a very simple behavior, you can measure how the mouse freezes as an indication of fear,” Li said. “You can then use a method to disrupt the amygdala to see if the animal becomes deficient in learning fear. And that indeed confirmed that the amygdala was important for fear learning.”
From these findings grew hypotheses that maybe the dysfunction of the amygdala was complicit in human disorders involving fear-learning, like anxiety disorders. PTSD falls under this umbrella of anxiety disorders, where an excess of uncontrollable fear memory spills over into a patient’s regular life, making them fearful and on-edge even in safe situations. In examining the brain images of anxiety-afflicted animals and humans, Li and fellow neuroscience researchers saw that the amygdala is a structure that shows consistent change and hyperactivity.
Using animal models, Li and his lab have been able to delve deep into the amygdala to see precisely where in the circuits of different cell types change is occurring in the event of abnormal activities.
In May last year, Li and his colleagues identified a neural circuit in the amygdala that gives rise to anxiety. The team showed that when they tweaked a neuron called SOM+ in the amygdala, they were able to heighten or ameliorate displays of anxiety in mice. The SOM+ neuron has been found in previous research to be implicated in learned fear responses. In addition, it’s also tethered to a gene called Erbb4, which has been associated with bipolar disorder and schizophrenia.
When we start to understand the diseases, we start to be able to talk about it, to communicate with the public.”
CSHL Professor Bo Li
This research contributes to a growing body of knowledge regarding different cell types in the amygdala, how they are changed during an anxiety disorder, and what other areas in the brain they are influencing. Currently, work is underway in the Li lab to see if chemically or genetically altering certain cells in this circuit can be helpful in alleviating anxiety-related behaviors in neurotic mice.
In other developments in the anxiety research field, scientists from Boston University found that circuits run systematically through the amygdala and the hippocampus (the memory center of the brain), to encode and respond to learned fears. Last year, Columbia University researchers found a hippocampal-hypothalamic circuit that is active when a mice experiences innate fear, like looking over a tall ledge or being vulnerable in an open field. With both learned fear and innate fear in rodents, there have been several studies in the past few years delineating a number of places in the brain that are crucial for the process of expressing defensive behavior. In those parts of the brain, a number of specific neurons and circuits have been linked directly to anxiety-related behaviors. Each new discovery colors in a more complete image of the brain. And as these different pathways are mapped out, scientists are starting to see how interconnected different cells and circuits are.
“There has been evidence that innate fear and learned fear use a common circuit, because if you destroy the amygdala, both innate fear and also learned fear are perturbed,” Li said. “That suggests that the amygdala may be the common pathway for mediating both the innate fear and also learned fear. However, that doesn’t mean that innate fear and learned fear use exactly the same neural circuits. For example, the superior colliculus (a part of the brain) is involved in the innate fear–like when a predator like an eagle tries to capture a mouse.” Knowledge of innate fear is a unique feature that the animals are born with, and the superior colliculus is critical for modulating that. But superior colliculus is not known for the classical fear conditioning that scientists study, otherwise known as learned fear.
It appears that at each processing stage along the way, the brain gets a slightly different piece of information about the structure of threats in the world. And what the scientific community studying anxiety has in front of them right now resembles an incomplete puzzle of intersecting and entangled pathways and circuits. “There is some overlapping, but also dissociation between the two fear circuits. There are new studies that are starting to reveal that these two circuit also talk to each other. They don’t operate independently. But how precisely they coordinate control in different aspects of fear, is still being studied,” Li said.
Motivation deprivation
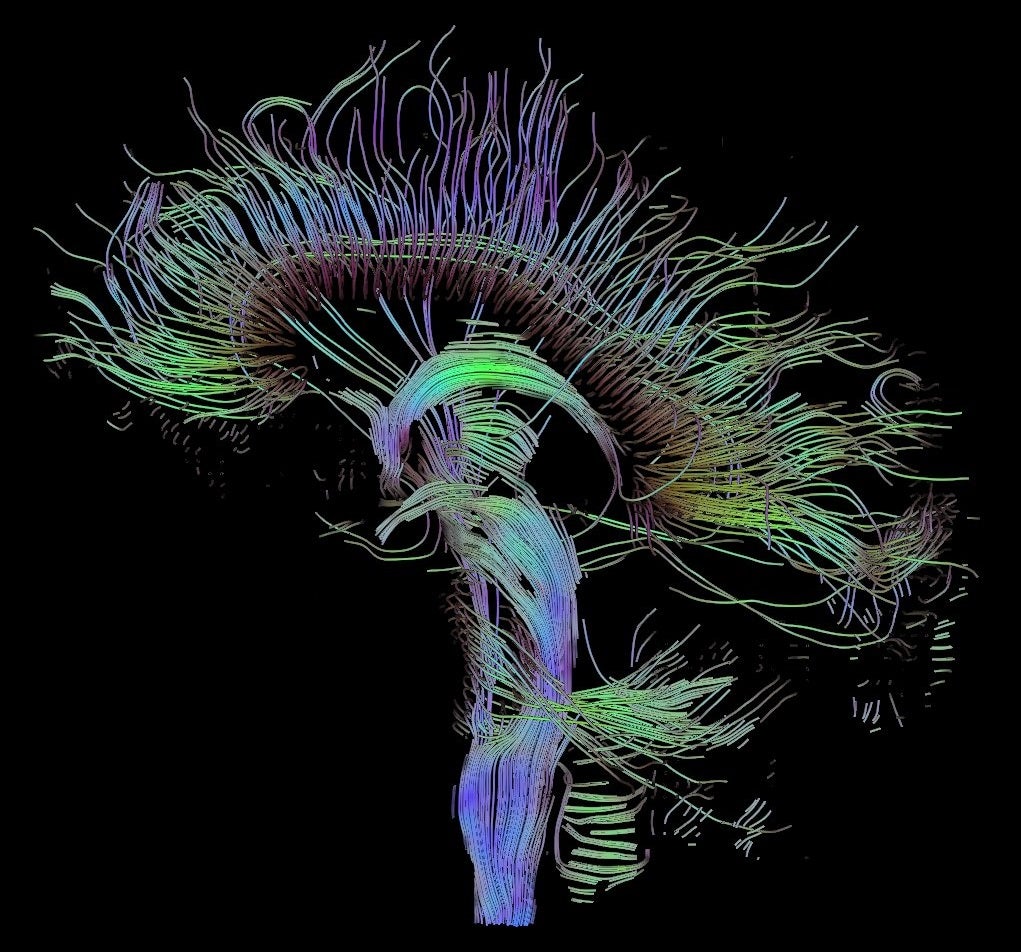
Li also studies disorders of depression, examining circuits that control motivation, which run through a lesser known structure in the brain called the lateral habenula (LHb). Hyperactivity in the lateral habenula contributes to the development of depression-like behavior in animals and humans such as decreased motivation to seek reward or increased sensitivity to punishment, according to Li.
But, he said, there is one small hitch with researching the LHb.
It’s very difficult to study this structure precisely, not only because it’s small but also because mouse genetics has not allowed researchers to target cells in the lateral habenula very accurately. He has tried to bypass this problem by studying the structures that work around the lateral habenula, and filling in the blank with what happens in that region by observing cell signal inputs and outputs.
“So we’ve been targeting two major synapses that input onto the habenula. One is from the ventral pallidum, which has been long hypothesized to be important for motivation, and the other is the dorsal striatum,” Li explained. “We found two cell types in the ventral pallidum that control the habenula: one promotes reward, the other promotes punishment.” He postulates that in a disease such as depression, the balance between the two inputs may have changed.
The whole is more complex than just the sum of its parts
“Eventually all brain structures connect to each other in some way, mostly indirectly, or are one, two or three synapses away from connecting,” said Li. Depression and anxiety are thought to run in antiparallel systems, sharing the same path but performing opposing tasks, and Li has found some evidence to support that claim. “There are indirect connection between these two system, but we have also found a direct connection between the amygdala and the basal ganglia. We are trying to understand how the two system actually interact with each other.”
If you step back to look at the big picture, all these different systems involved in different types of mental illnesses share many commonalities. That’s why Li thinks that putting the terminologies anxiety, depression, bipolar disorder, and schizophrenia into these separate, neat little boxes is an outdated way of classifying mental illness. As the neuroscience community understands more and more, those terminologies become insufficient because “in each of these disease groups, each patient may have very different phenotypes; and also the cause of the phenotypes can be quite different,” Li said.
“I think there is a consensus in neuroscience research to try to understand individual behavior change in a particular patient. For example, when we’re talking about schizophrenia, one affected person may express psychosis, or lack of motivation, or anxiety. Each of these problems may be traced back to the circuit that we study that control each of those behaviors. In schizophrenia, psychosis could be related to dopamine, to the striatum, but anxiety may be related to the amygdala,” he added.
Another issue is that many patients experiencing mental disorders have comorbidities, which means there are multiple conditions that occur simultaneously. “Saying this is schizophrenia, this is bipolar disorder, and this is depression may not be enough,” Li argued. “A particular behavior change may be involved in multiple diseases. We have to study how each of these circuits controls particular behaviors, and how these neural-circuits are shared between different diseases.”
Walk through of online 3D brain app by the DNA Learning Center and Cold Spring Harbor Laboratory
In a research area with lots of moving parts, one research lab is not an island. Li attributes many successes in mental illness research to the spectacularly symbiotic environment of the neuroscience community. His own research, he said, has benefitted immensely from the many fantastic collaborations he’s had with researchers like Karl Deisseroth, Ed Boyden, and CSHL Professor Josh Huang. And as the biological underpinnings of mental disorders are slowly brought to light through science, Li is beginning to see a lot of the stigma slowly be chipped away.
“People start to understand the cause of certain disease-related phenotypes. They also start to understand that it’s a part of an experience of the whole population, that we all have,” Li said.
Actress Kirsten Dunst’s character once famously said in the 2004 movie Eternal Sunshine of the Spotless Mind that “adults are like this mess of sadness and phobias.” Although that notion may hold true to some extent for all of us, for many, those feelings can be on a more extreme end of the continuum.
Different personalities are prone to different stresses. In a lifetime, you maybe affected by one of these diseases, such as depression or bipolar disorder, the two disorders with the highest lifetime prevalency (greater than 15%), and sometimes, more than once in a lifetime.
In 2017, there were an estimated 46.6 million adults aged 18 or older in the United States who experienced some form of mental illness, according to the National Institute of Mental Health. In that same year, approximately 11.6 million adults experienced a serious mental illness that substantially interfered with, or limits, one or more major life activities.
“When we start to understand the diseases, we start to be able to talk about it, to communicate with the public. And that then also helps neuroscience move forward,” said Li. “The next thing is how to use this knowledge to really influence clinical treatments and diagnosis. This is something we are trying to push.”
Li looks back at the inception of his curiosity as an inquisitive, young medical student. “Back then, I couldn’t imagine that this can happen, that we can reach this stage,” he reflects. “But right now, together with basic animal research, technology, and genetics, everything is starting to come together and move forward faster. It’s very exciting.”
[Think you know your way around a disordered brain? Test your neuroscience knowledge in this short trivia.]Written by: Charlotte Hu, Content Developer/Communicator | publicaffairs@cshl.edu | 516-367-8455
About
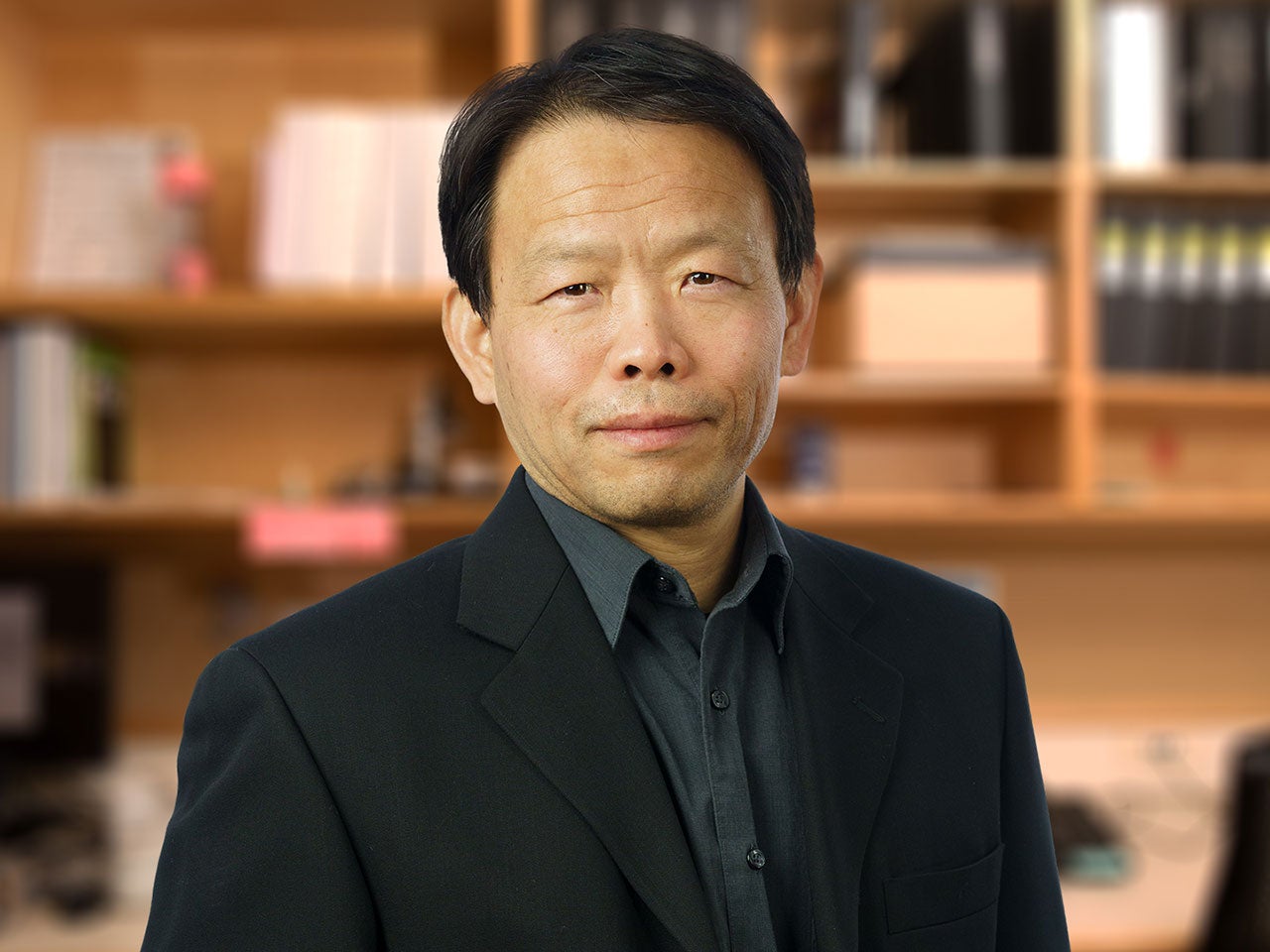
Bo Li
Professor
Robert Lourie Professor of Neuroscience
Ph.D., The University of British Columbia, 2003