A small, hairless rodent has mastered the secrets of the universe. It rarely gets cancer, resists signs of aging, doesn’t experience much pain, survives for 18 minutes without oxygen, and lives nearly 20 times longer than most small rodents.
In the past few years, despite being one of the most odd-looking creatures on Earth, the naked mole rat’s meteoric rise to scientific fame as a new model organism—which are non-human animals used in the laboratory to help scientists understand basic biology and physiology—has even captured the attention of tech giants like Google. In fact, Google spun out a company called Calico Labs that’s dedicated to investigating how this peculiar creature conquers aging, cancer and pain.
The octopus is also on its way to become a new model organism. It has the largest brain-to-body ratio of any invertebrates, and is incredibly smart. By making them a model organism, researchers seek to understand the evolution of intelligence between primates and a species alien from them.
The variety of organisms studied is currently undergoing a massive expansion, spurred by the curiosity to uncover differences and similarities in the diverse portfolio of life. The efficiency of genome sequencing, as well as the widespread application of selectively altering gene expression, is allowing biologists to expand their research to include the latest evolutionary context. CRISPR, a new technique that can quickly and cheaply modify entire genomes, is fueling this revolution.
Animals of all types can teach scientists about life and the world we inhabit. For that reason, scientists have been entranced by the mysteries of the animal world for decades. Technology developments over the years have expanded the capability of how closely scientists can study living organisms. Better animal models and tools help scientists translate and study human disease and improve our understanding about how living systems function.
The process of science is non-linear. Independent discoveries are often segmented across time as technology and knowledge allow, and stitched together into more comprehensive pictures after. New technologies may not reach their full utility until decades later. Likewise, questions of science are revisited when appropriate tools to answer them come into existence. The specialty fields of genetics and neuroscience present a curious case study for why a diverse portfolio of animals can be effective in advancing basic science.
Marine life gives birth to a new lab
Evolution has created well over 8 million species on our planet, each adapting specialized skills and functions that help them survive in their natural environment. Throughout history, scientists have strategically used organisms with specialized traits to study the inner workings of cells and organs.
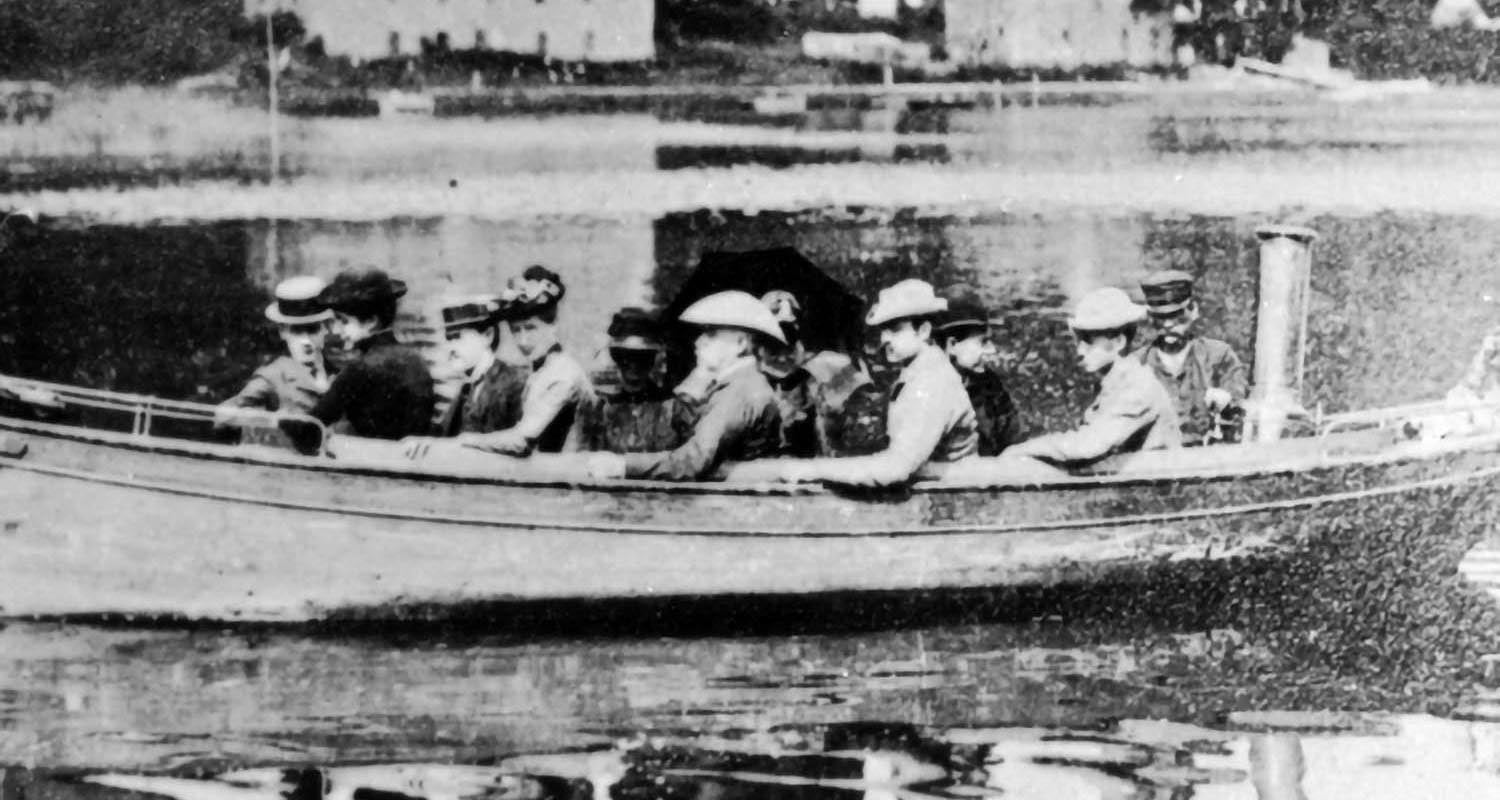
The field of experimental biology evolved from a generalized form of observational biology, where researchers focused on the parts of animals that were visible to the naked eye. To chart the changes in the uses of animals in research is to chart the change in the way researchers conduct scientific experiments. The evolution of Cold Spring Harbor Laboratory from a natural-science focused marine observatory to a premiere genetics research institute is a good example.
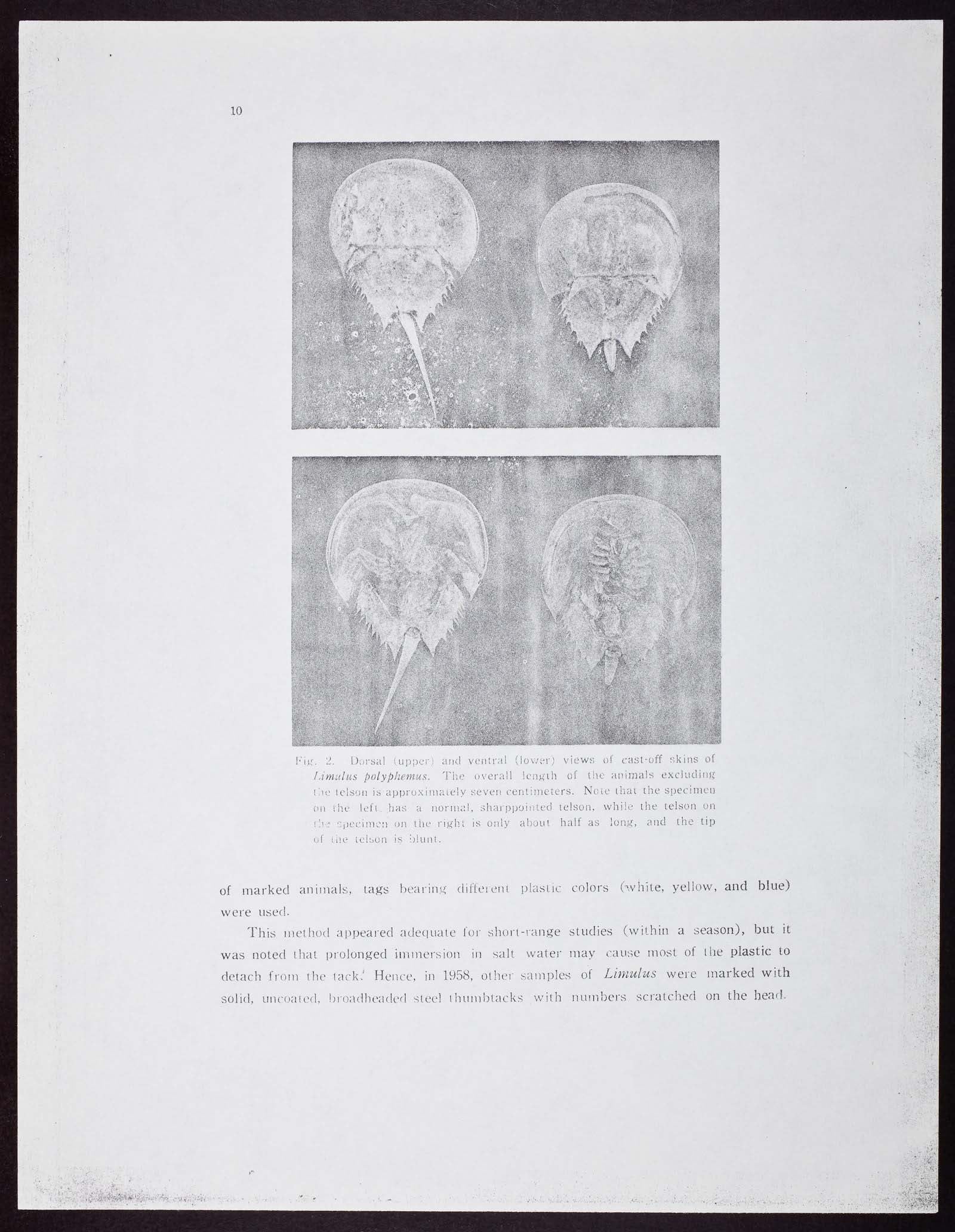
The first iteration of the Lab was born in a fish hatchery as a seaside biology school for teachers.
The Biological Laboratory, as it was named, was then a division of the Brooklyn Institute of Arts and Sciences. It opened in 1890 as a summer school and a natural history haven, offering courses about the local habitats and primers on the major types of organisms. The horseshoe crab, which was bountiful in the harbor, was a creature of great interest to the students, who often analyzed its anatomy and population variations.
The school sat at the precipice of a new era in science. Its opening came at an opportune time, as science became increasingly popularized in the 19th and 20th centuries. Governments started funding research, and science shifted from being a private interest to a formal profession.
Cold Spring Harbor Laboratory’s birth also coincided with the infancy of a new field of science. This period saw Charles Darwin and Gregor Mendel break new ground with their findings on genetics and evolution. Their theories and observations of heredity opened a cabinet of curiosities that invited follow-up questions that greatly influenced research at the Lab.
Animal models gain importance
Technology at the time was crude compared to the refined apparatus found in laboratories today. The lab in the fish hatchery was supplied with aquariums, hatching troughs, glassware, dissecting instruments, microtomes to cut biological materials into thin slices, microscopes and photography equipment. A few small boats floating outside the lab-made trips into the harbor to collect marine specimens.
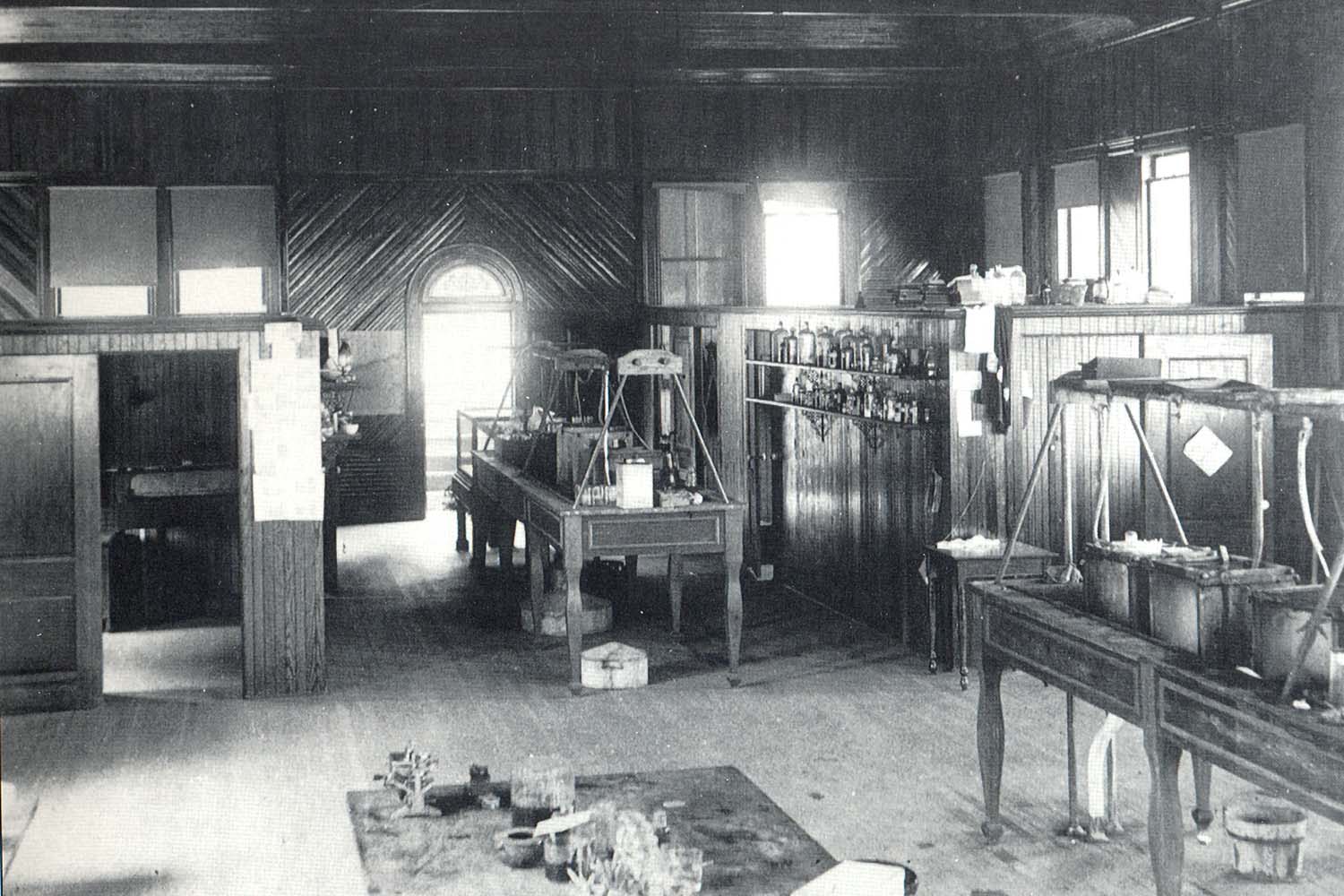
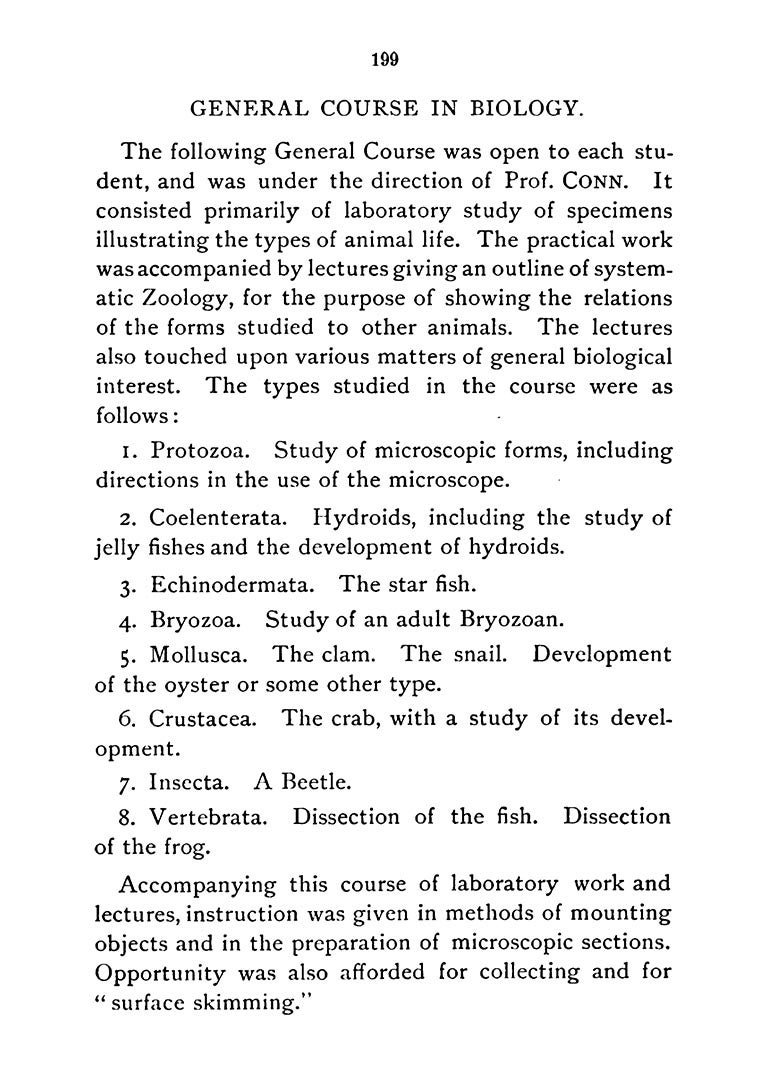
With this basic technology, students were trained in the fundamental techniques of natural history, embryology, and anatomy. They had to collect, microscope, dissect, and stain all their samples. A lot of the science done at the time was on features and changes at the macro-level. There was no way to delve into or instigate changes at the micro-level. The powerful microscopes of the 19th century could see down to the individual chromosomes in the cell during mitosis, but could not discern their DNA subunits. Students at the lab could see the physical development of an embryo but not the underlying chemical and biological processes supporting it.
By the turn of the 20th century, the science world shifted from zoology and botany (descriptive disciplines) to physiology and morphology (experimental and analytical disciplines). In 1891, the lab introduced “quantitative biology,” which integrated physics and chemistry into biology. It was a way to unravel patterns and correlations underlying series of observations. It also encouraged more statistical, analytical approaches to research, rather than being merely descriptive. Studies in genetics and evolution profited immensely from the quantification of science. Animal models were starting to be used in a concise fashion, which consolidated the range of organisms studied.
In 1903, the Carnegie Institution of Washington decided to establish a Department of Experimental Biology, consisting of a Station for Experimental Evolution at Cold Spring Harbor. The new research lab opened in June 1904.
Gregor Mendel’s demonstration with pea plants established some basic rules of trait inheritance and presented a fundamental model for experimental genetics. While some scientists like George Shull, Anne Lutz, and later, Barbara McClintock followed in his steps and went on to make landmark findings in plant genetics, other scientists at the lab wanted to replicate these results in animals.
Charles Davenport, the first director of the Station for Experimental Evolution (later known as the Department of Genetics), was very hands-on with his experiments. He would describe the measurements and statistical analyses of physical traits to differentiate natural groups of organisms in local habitats that supported his theories of adaptation. He also piloted a series of breeding experiments.
Animal genetics explode
Animal genetics soon took on a life of its own at the lab. Davenport bred poultry, canaries, cats, sheep, goats, insects, plants, mice, rats, guinea pigs and rabbits. He published many papers on coat color in mice, origin of black sheep, heredity of traits in poultry and the transplantation of ovaries between hens.
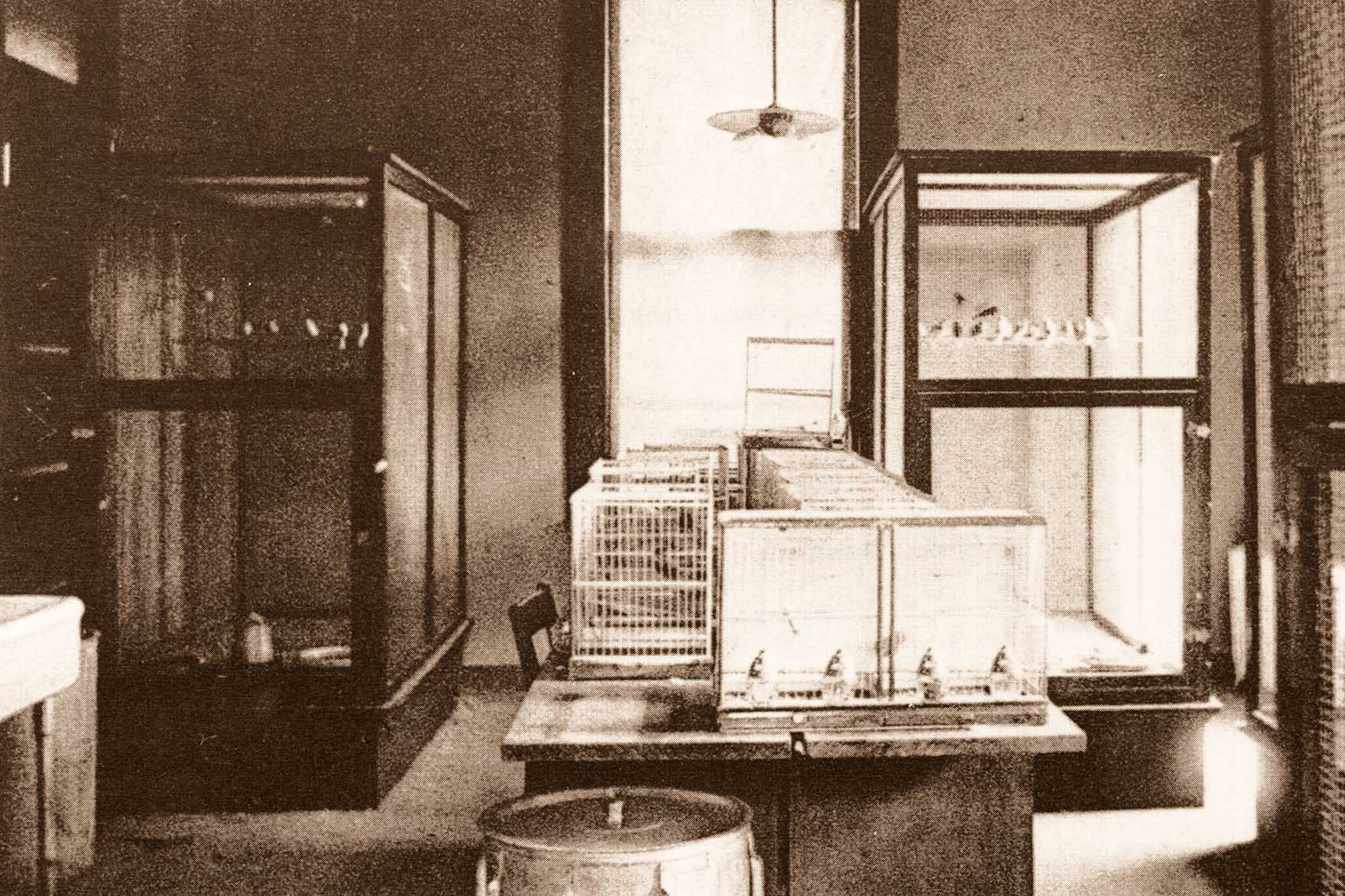
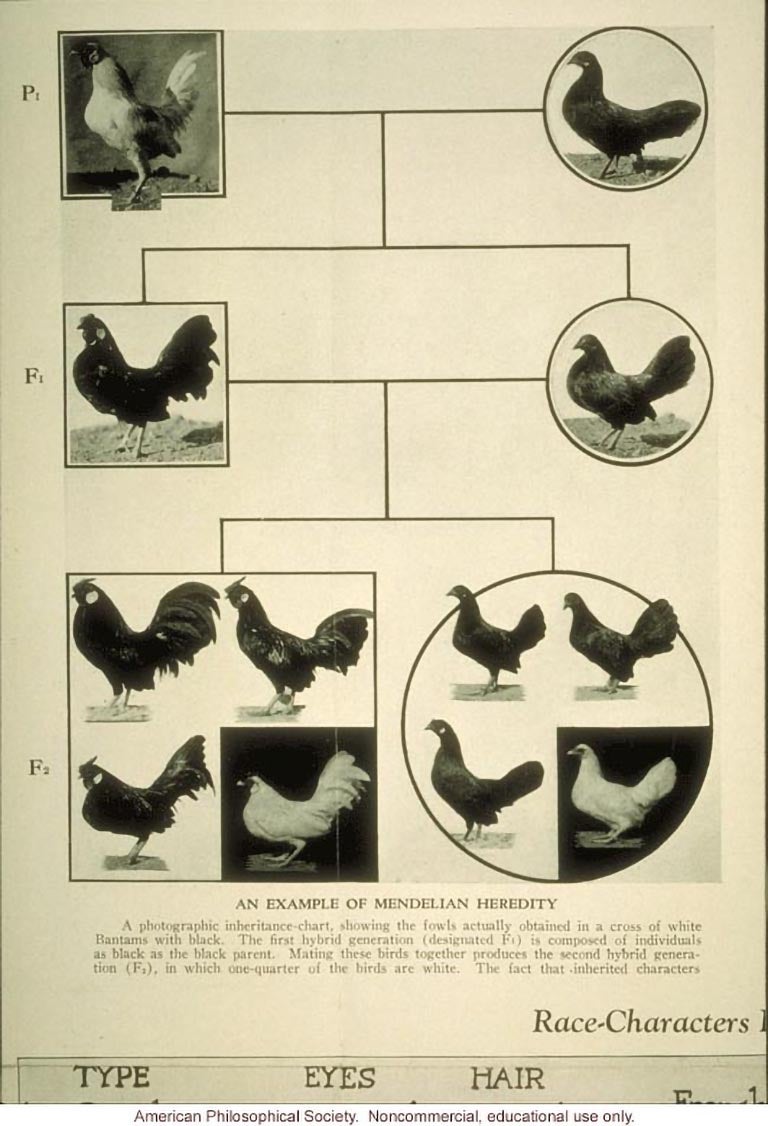
Around 1920, genetics became the predominant research theme at the lab, three years after it was incorporated into the Brooklyn Institute of Arts and Sciences. At this point, the lab had two large pigeon houses, a wide variety of animals, fields and greenhouses filled with experimental crops, and the “cave” housing subterranean creatures. The cave was courtesy of Arthur Banta, an investigator at the Lab who from 1910-1930 created an artificial environment under the lab to study whether animals lost traits from disuse. He started with a zoo of species and ultimately zeroed in on the tadpoles of tiger salamanders (ambystoma tigrinum). He found that the normally dark animals grew up pale when raised in the cave, but could not say if such an effect was heritable.
Using Mendel’s rudimentary experimental techniques, some genetics researchers sought to translate pea plant heredity to animal models. Drosophila melanogaster, or the fruit fly, became one of the first animal models to test this theory, because they had a simple genome (only have four pairs of chromosomes) and a short-life cycle.
Flies started popping up in genetics research labs all across the country at the beginning of the 1900s. CSHL entomologist Frank Lutz was one of the first to use these animal models for experimental evolution. He studied fruit flies in controlled settings to see whether inherited characters influenced development. He reared over 70 generations of fruit flies to observe the inheritance of abnormal wings. He found that certain traits were dominant while others are recessive, and that temperature and environment, as well as sexual selection, played a role in heredity. He introduced fruit flies to colleague Thomas Hunt Morgan, who went on to win a Nobel Prize for his work with Drosophila genetics. Morgan furthered fruit fly genetics by integrating discoveries from embryo development and cell biology observations into his research.
Morgan’s work carried back over to CSHL when Charles Metz, a former graduate student of his, ultimately found 10-11 chromosomal complexes in fruit flies by 1916, and discerned a pattern for chromosome pairing during meiosis, a special type of cell division. The invention and popularization of tools like x-ray crystallography in the 1910s allowed scientists to make out atomic and molecular structures of proteins and other biological molecules. It also allowed geneticists to zoom in further on the chromosome. Milislav Demerec made CSHL a center for Drosophila genetics in the 1930s. They breed fast, and each gene had mutable, widespread effects to the fly’s physiology. Demerec penned a number of guides and publications to aid investigators interested in working with fruit flies. He established a Drosophila stock center at CSHL in 1933. But he also was open to new organisms and tools if they offered him better opportunities for genetic analysis.
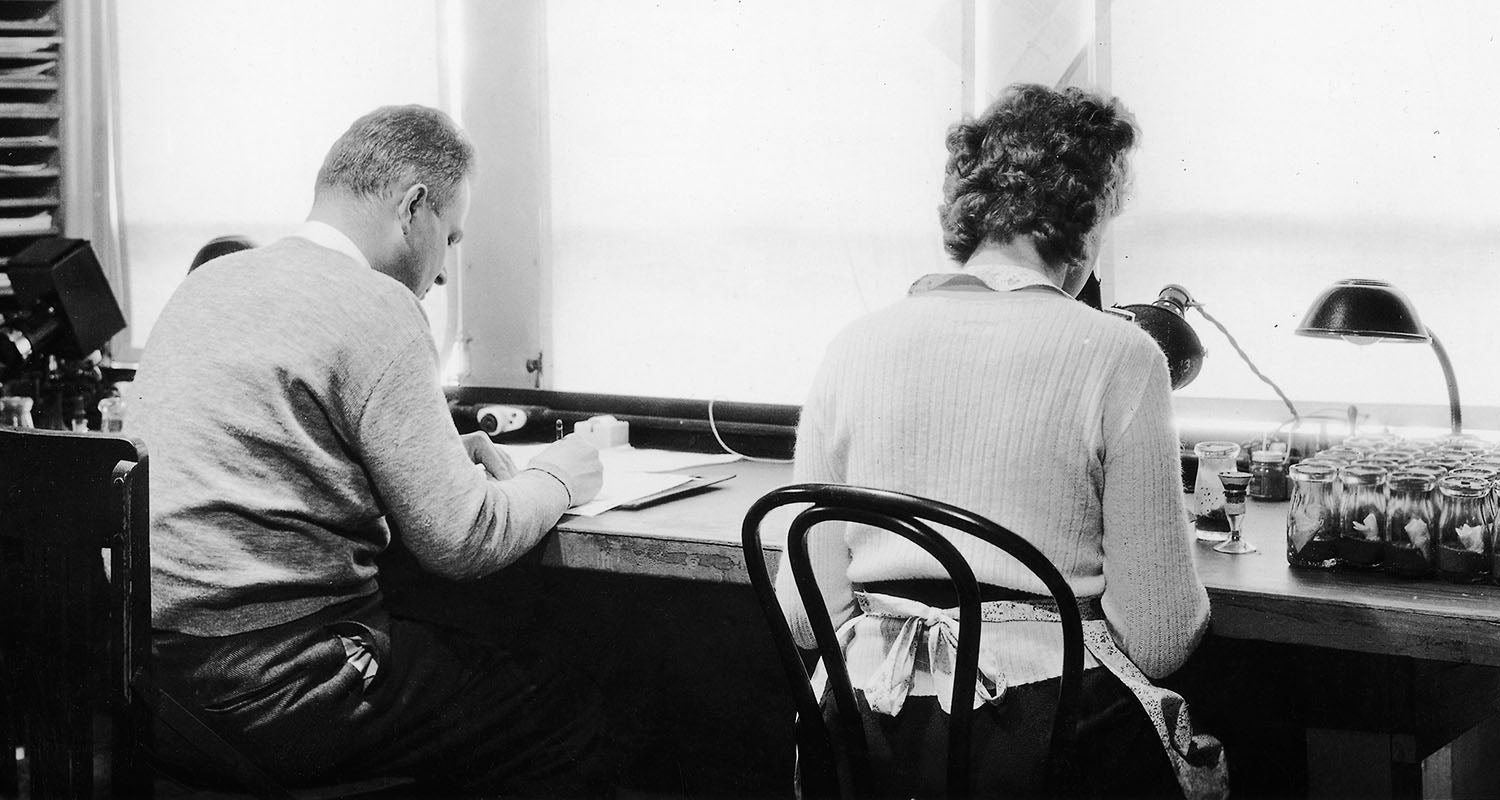
The series of genetics experiments and findings first established in fruit flies set the groundwork for the transformation of biology into an experimental science. And because evolution has conserved a lot of the same metabolic and developmental pathway across many different species of animals, researchers were able to use these primitive models to examine how complex animals worked.
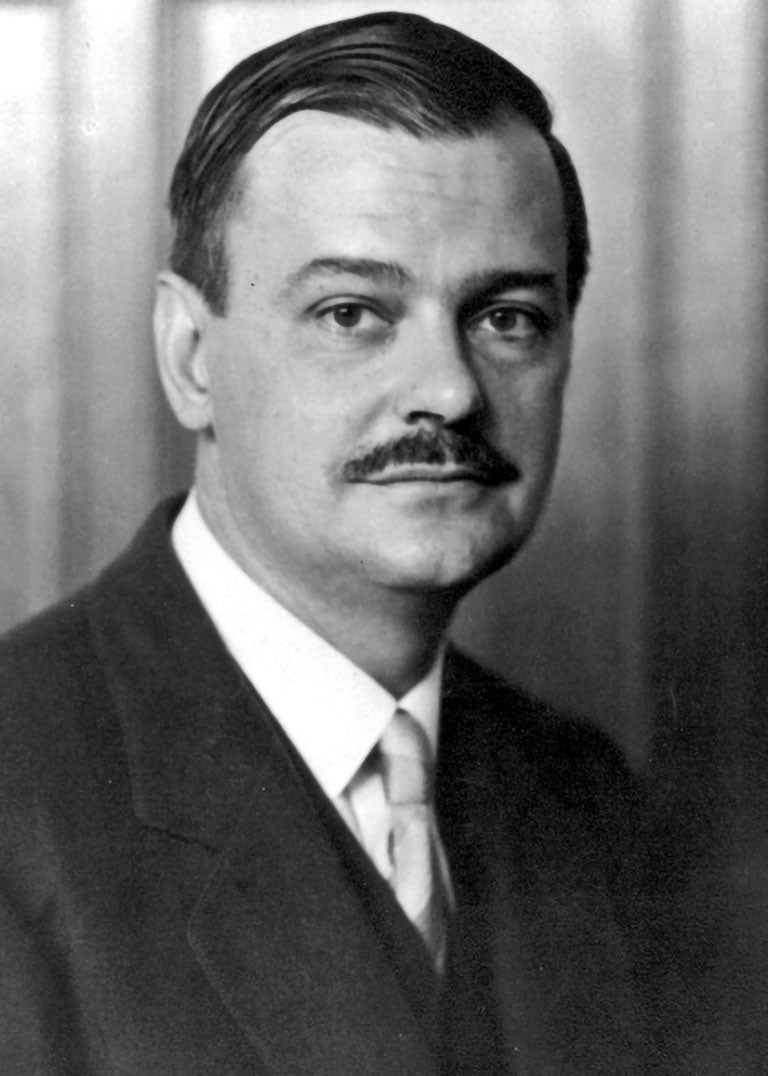
Up until the 1920s, scientists have mostly been observers of curated breeding experiments. Clarence Little, a cancer geneticist, tried a very different approach in 1919 using the Japanese waltzing mouse, which had a neurological disorder due to inbreeding. He transplanted tumor tissues from one mouse to another of the same strain and observed that certain mouse lines were resistant to developing tumors when injected with sarcoma particulates while others went unaffected. His findings expanded research into organ transplant rejection, in which genetics played a role. His experiments also formed the basis for the use of genetically identical, inbred mouse lines for lab testing. These mice standardized genetics experiments and allowed scientists to study the effects of specific genes on disease unmuddled by genetic variability across different animals.
Unlocking the mouse genome allowed researchers to graduate to a complex mammalian model organism that shared a similar circulatory system and more parallel correlates with humans. It paved the way for understanding the human genome, and also for the recreation of human disease models in mice.
Little eventually founded the Jackson Laboratory in Maine, which houses and sources the largest collection of inbred mice to laboratories all across the world. It’s now the foremost center for studying mouse genetics.
Specialties converge, animal models expand
Outside of the burgeoning field of genetics, CSHL also housed scientists from a number of other specialty fields. One of them was endocrinologist and pituitary gland Oscar Riddle, who in 1920 experimented with hormones in pigeons. In 1932, after investigating the crop milk of pigeons, he isolated prolactin, the hormone that tells the body to make breast milk, from the tissue extracts of anterior pituitary gland.
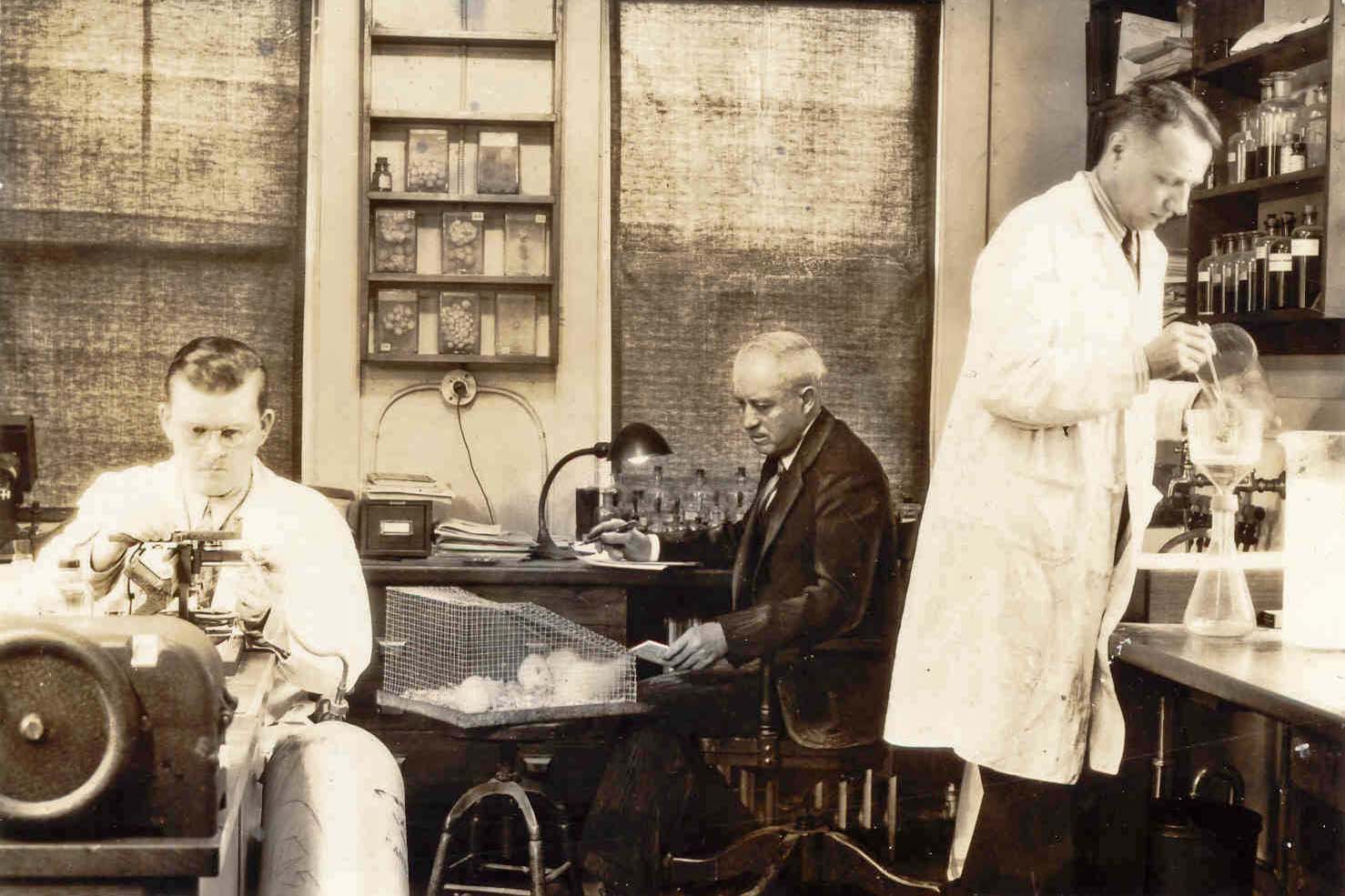
JJ Pfiffner, another endocrinologist from the lab, worked with a colleague from Princeton University to isolate the adrenal cortical hormone, which maintained and regulated the circulation of fluids in the vascular system. This hormone was later used to help patients with Addison’s disease, a disorder that occurs when the body doesn’t produce enough of a certain hormone.
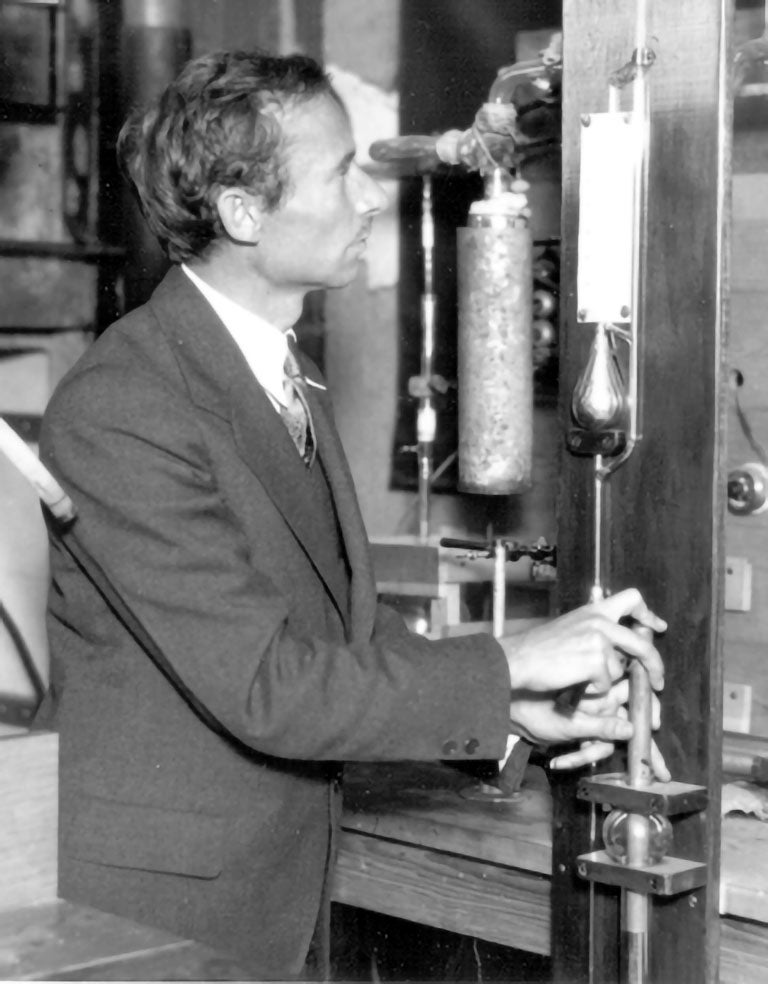
The Lab during this time experienced other changes that would establish its future direction. It raised admission standards in the summer program for aspiring researchers; added new courses, such as animal bionomics, ecology, and evolution; and began independent research. Reginald Harris, who served as the Lab’s director from 1924-1936, established a program in biophysics and solidified the Lab as year-round research institution. Scientists now used cutting-edge tools such as electrodes, voltmeters and conductivity meters to explore the electrical basis of nerve cell activity and how they passed messages along nerve fibers. The program recruited physicist Hugo Fricke as the lab’s first full-time research investigator. Fricke, who had previously studied the effect of x-ray on cells and the properties of the cell membrane, applied physics to biological and medical problems. He tried to use his findings to decode the structure of cell membranes. This meant breaking into the realm of physiology, specifically how hormones mechanistically targeted specific cells. Fricke worked mainly with rabbits, studying their tissues and components in their blood. Rabbits are now commonly used in laboratories for the production of antibodies, a protein produced by plasma cells and used by the immune system to neutralize pathogens such as bacteria and viruses.
Cats, squids and worms contribute to neuroscience
Around this time, as scientists started more intricately studying the cells and their makeup, modern neuroscience, or science about the brain, came of age. It was a specialty field that concentrated on delving into the biological roots of less grandiose and bizarre theories from psychology. At first, it was largely an observation-based study fueled by guesswork and serendipity. The focus shifted to being more mechanistic when more complex technology evolved and better animal models became available. Neuroscience became fundamentally different from psychology in that it cared about physical and testable cause and effect, and about how cells in the brain functioned and worked with one another.
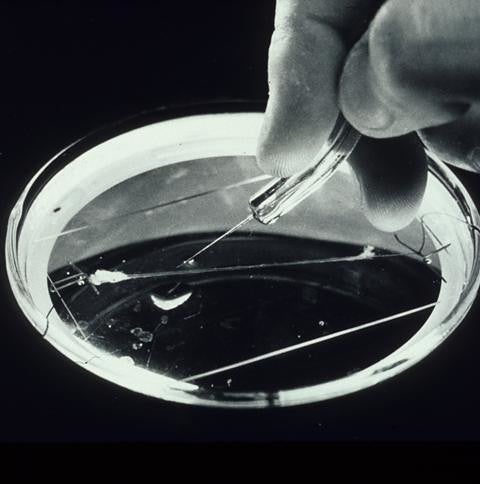
Camillo Golgi and Santiago Ramón y Cajal in the 1890s proved the existence of neurons, the basic building blocks of brains from staining nerve cells from cats, who had complex memory, cognitive, and sensory systems. Cat brains were large enough to peer into and observe with a primitive microscope.
Electron microscopes, invented in 1933, were powerful enough for researchers to peer into neurons in the brain of simple organisms and trace how they were linked. This inspired the effort to create a connectome, a map of all the neurons and their pathways across the brain.
The next landmark discovery in neuroscience came in the 1950s, when scientists Alan Hodgkins and Andrew Huxley from the University of Cambridge figured out how neurons actually fired, using squids endowed with giant neurons to catalyze their discovery.
Drosophila and C. elegans (a translucent roundworm) became the chief model organisms in neuroscience research. Sydney Brenner, a CSHL course attendee and friend of the Lab, spearheaded the effort to map the connectome of C.elegans in the 1970s. The project was finally completed in July 2019. The ease of doing neuroscience, the solidification of the basic biology behind how the brain worked, the ability of recombinant DNA procedures to manipulate the brain at a molecular level, and an influx of funding to support various neuroscience programs, all led up to the opening of a neuroscience research center (comprised of the Beckman Laboratory, Dolan Hall, Hazen Tower, Hughes Teaching Laboratories, and the Keck Structural Biology Laboratory) on campus in 1991.
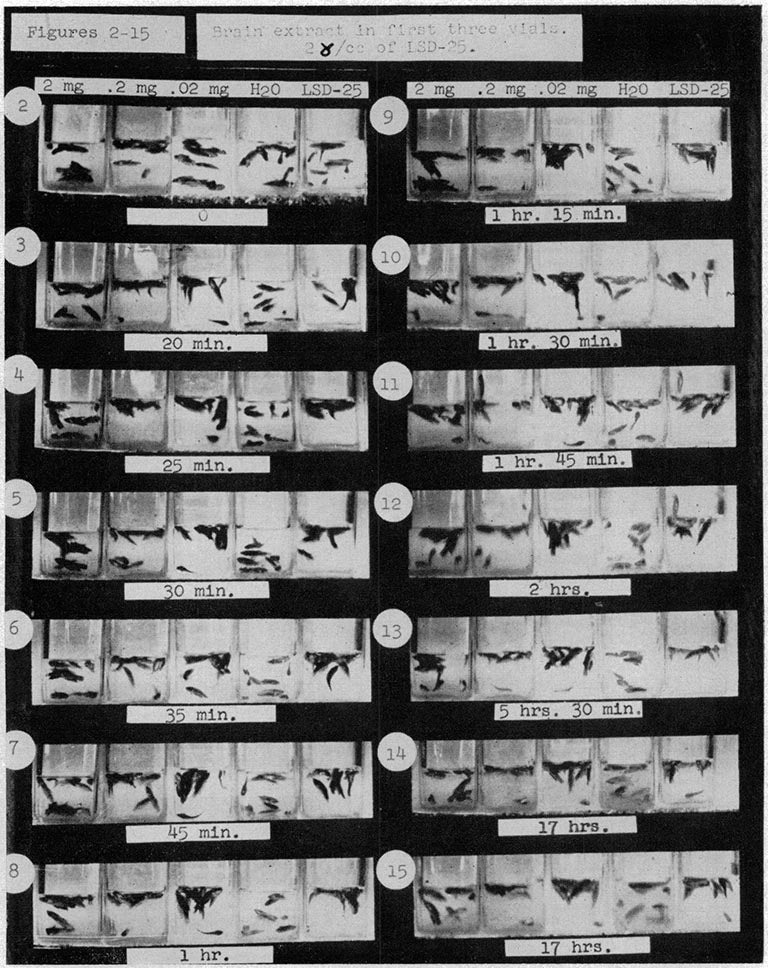
Even before the formal introduction of neuroscience as a research program, CSHL researchers started to dabble with psychedelic experiments. In the 1950s, CSHL scientist Harold Abramson began studying the effects of LSD on guinea pig brains. He also tested water fleas, Drosophila, the mystery snail, and the Siamese fighting fish to find causes and potential treatments for psychosis. It turned out he had been covertly doing work for the CIA as a part of its MKULTRA program to understand how mind-controlling drugs worked.
By the late 1900s, technology allowed scientists to do more with less. Researchers used mice to stand in for many earlier neuroscience models. Mice became the predominant model organism, as the lab gradually retired fruit flies and other elementary animal models. The introduction of transgenic mice, or mice who had their core DNA altered, in 1980 broadened scientific knowledge on a range of human diseases, from cancer to neurological disorders. Around this time, federal agencies developed the Guide for the Care and Use of Laboratory Animals as an ethical infrastructure to ensure that research animals were properly used and humanely treated.
Read more: Animal care and research at CSHL
Today, CSHL houses mice, rats, and voles from the rodent family. The 600 researchers and technicians on-campus use these animals to study dynamic problems in cancer, neuroscience, quantitative biology, plant biology, bioinformatics & genomics. The mice are used by all scientists, especially by the cancer biologists, who account for 49% of the total researchers on campus. They study how mice develop spontaneous forms of cancer as well as how they fare under genetically induced cancers. The rats and voles are primarily used by behavioral neuroscientists who observe how animals use different types of sensory input to make decisions. Many scientists at the lab now study an amalgamation of disciplines (such as genetics and neuroscience, or genetics and cancer). Some neuroscience labs use the large egg cells of frogs and axolotls to study embryonic neural development and neuron signaling.
A hospital for mice
The Lab is ever-changing in what it studies and how its researchers study it. Cancer from the time Clarence Little studied it in the 1920s is very different from how cancer is studied by the CSHL Cancer Center today.
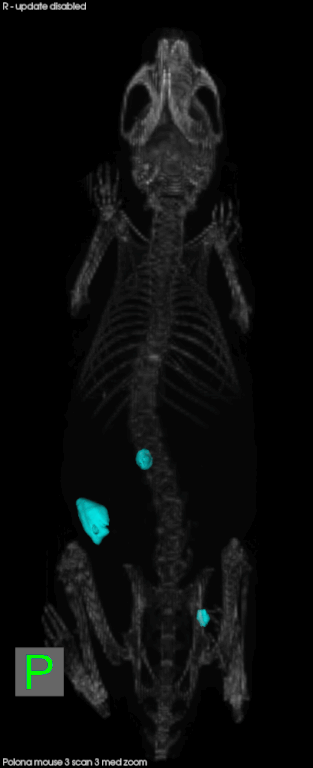
Later this year, CSHL will open the Preclinical Therapeutics and Imaging (PTI) Facility with the goal of delivering validated drug candidates to industry. It will allow scientists to conduct drug testing in animal models for cancer in a way that mimics cancer in human patients. Mice, for example, will develop pancreatic cancer in their pancreas, rather than having a transplanted human tumor on their skin. Scientists can also compare several different tumors in one animal.
Everything in the facility is scaled down to accommodate mice patients. They can undergo CTs, MRIs, micro CTs, pet CTs, and ultrasounds just like at human hospitals. There are also mice-specific surgical suites and diagnostic labs. In conjunction with better animal models for disease, technology has made it easier to follow the animal on its natural disease progress in a less-invasive, and human-like manner, and look at real-time effects of experimental therapeutics. The animal can serve as its own control, and it reduces the number of animals needed for a given experiment.
“About 40 years of fundamental cancer research has brought us to the stage where rational approaches to cancer therapy are now possible,” Bruce Stillman, President and CEO of the CSHL, wrote of the facility’s purpose. “I look forward to the day, in the not-too-distant future, when our neuroscientists will likewise apply their knowledge of how the brain works to use the facility to advance treatments of the numerous neurological and psychiatric disorders.”
Written by: Charlotte Hu, Content Developer/Communicator | publicaffairs@cshl.edu | 516-367-8455