Why is histone H3.3 associated with active genes, but histone H3.1 is not?
Cold Spring Harbor, NY — Every cell in our body has exactly the same DNA, yet every cell is different. A cell’s identity is determined by the subset of genes that it activates. But how does a cell know which genes to turn off and which to turn on? While the genetic code carried in our DNA provides instructions for cells to manufacture specific proteins, it is a second code that determines which genes are in fact activated in particular cell types.
This second code is carried by proteins that attach to DNA. The code-carrying proteins are called histones. Today, researchers at Cold Spring Harbor Laboratory (CSHL) and colleagues publish research revealing a new layer of complexity in the histone code. They have found that the slightest variation in a single histone protein can have dramatic effects on how the genes encoded in our DNA are used.
Histones are vitally important because our genetic material is vast: every cell in the body has more than six feet of DNA bundled within a tiny nucleus, a space much smaller than can be seen with the naked eye. For such a massive amount of DNA to be compacted into a microscopic space, it must be wound tightly around spool-like assemblies of proteins. Each of those spools is made up of eight histone proteins. It takes millions of spools in every cell to bundle the entire genome.
Histone proteins are marked with chemical tags, such as methyl groups. The histone code consists of the patterns formed by such marks, across the full genome. The marks are sometimes called epigenetic marks. “Epi” means, literally, “above” the genome. The “second” code consisting of these marks provides instructions that cause cells to turn specific genes on or off.
There are numerous types of histones, and small variations in their structure enable them to perform distinct specialized functions. Scientists have found that one histone, known as H3, comes in two subtypes, called H3.1 and H3.3. These variants are found in very different places in the genome: version H3.1 is found only in parts of the genome where genes are not being activated; version H3.3 is present only in places where genes are active. Scientists have long wondered what it is about these two variants that accounts for their different associations with genes—H3.1 with inactive genes and H3.3 with active ones.
In a paper published today in Science, a team led by CSHL Professor and HHMI Investigator Robert Martienssen and Professor Jean-François Couture of the University of Ottawa announces that they have solved the mystery, exploiting unique aspects of plant genomes. They have discovered that a single amino acid difference in the structure of histone H3.3 enables it to serve as a kind of memory device for the cell, marking genes that need to remain active.
Martienssen, Couture, and Yannick Jacob, Ph.D., a postdoctoral fellow at CSHL and lead author on the paper, found that the key was a single epigenetic modification. The team, in collaboration with Professor Danny Reinberg from New York University, found that H3.1 can be modified with a methylation mark in a way that H3.3 cannot be. This chemical modification acts as a flag, signaling to the cell that genes in the vicinity should be inactive, or silent. “Our findings highlight the remarkable impact that subtle structural differences between histone H3 variants has in the global landscape of epigenetics,” says Couture.
This distinction is especially important when a cell copies its genetic material, which happens just prior to cell division. As the cell replicates its DNA, it must also preserve the epigenetic marks that delineate active and inactive areas of the genome. In fact, silencing machinery, which deposits methylation marks on H3.1, works in tandem with the replication machinery. “Because H3.3 can’t carry this modification, its presence on active genes allows them to escape silencing,” says Jacob. “In our research, we discovered a way for cells to protect active genes from silencing and preserve that memory through successive cellular generations.”
This study also has implications for how the genetic material is copied. “We have found that replication (how DNA copies itself) and transcription (how DNA is copied into RNA) are controlled by the same highly conserved histone. Thus, these most fundamental properties of the genetic material are regulated by our chromosomes,” says Martienssen.
Written by: Jaclyn Jansen, Science Writer | publicaffairs@cshl.edu | 516-367-8455
Funding
This work was supported by the Howard Hughes Medical Institute–Gordon and Betty Moore Foundation and by grants from the National Science Foundation; the National Institutes of Health; the Canadian Institute of Health Research; the Natural Sciences and Engineering Research Council of Canada; and an Ontario Early Research Award; a Canada Research Chair in Structural Biology and Epigenetics; and a Louis-Berlinguet postdoctoral fellowship from Fonds Québécois de la Recherche en Santé/Génome Québec.
Citation
“Selective Methylation of Histone H3 Variant H3.1 Regulates Heterochromatin Replication” appears in Science on March 14, 2014. The authors are: Yannick Jacob, Elisa Bergamin, Mark T. A. Donoghue, Vanessa Mongeon, Chantal LeBlanc, Philipp Voigt, Charles J. Underwood, Joseph S. Brunzelle, Scott D. Michaels, Danny Reinberg, Jean-François Couture, Robert A. Martienssen. The paper can be obtained online at: http://www.sciencemag.org
Principal Investigator
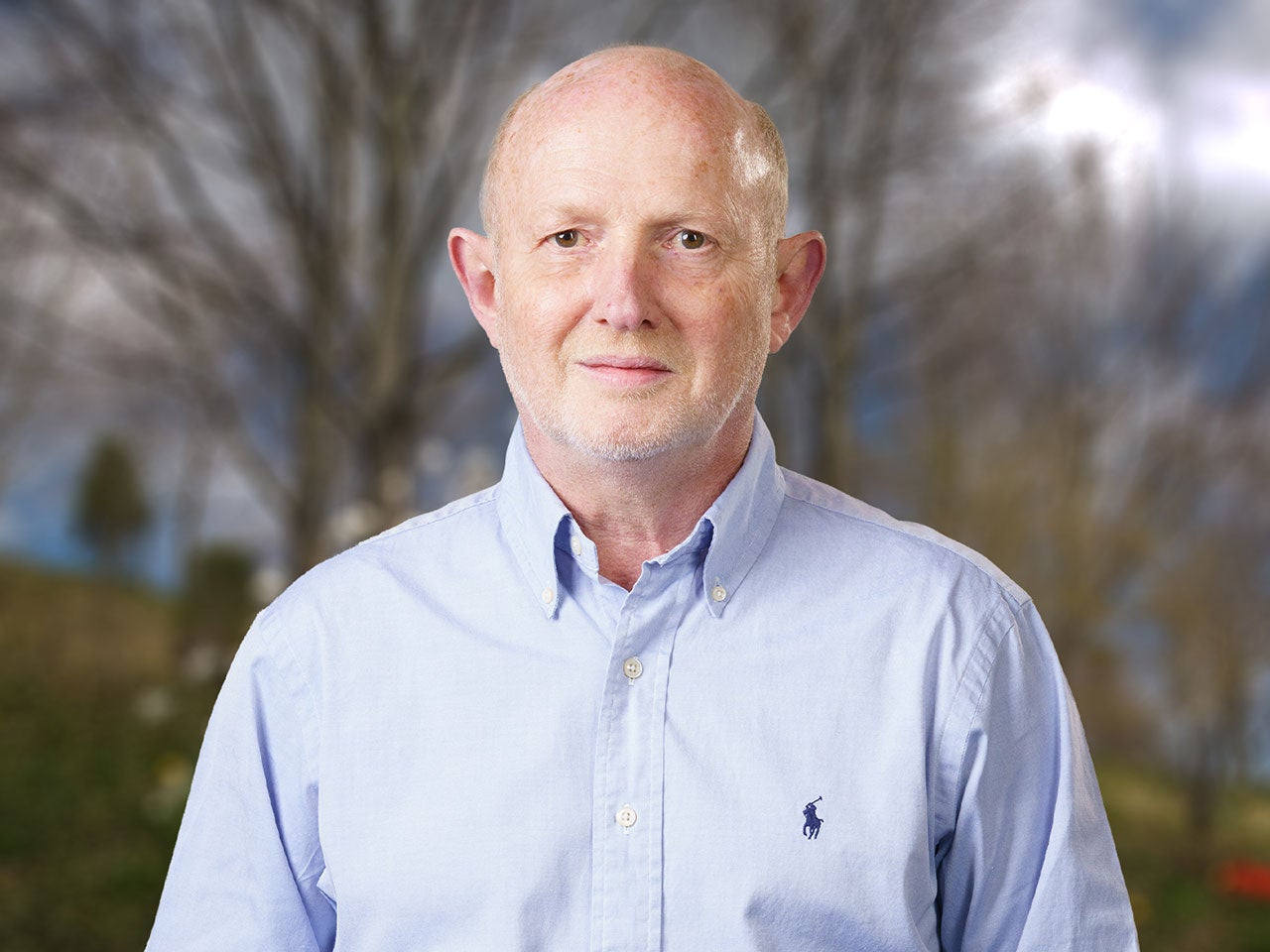
Rob Martienssen
Professor & HHMI Investigator
William J. Matheson Professor
Cancer Center Member
Ph.D., Cambridge University, 1986