Base Pairs podcast
As temperatures around the globe continue to rise, scientists are working hard to develop solutions that deal with the consequences of climate change, focusing on ways to slow or halt the trend. One significant hurdle is our dependence on fossil fuels like gasoline, coal, and petroleum. Researchers are working with a variety of biofuels that can power our planes, trains, and automobiles. Some biofuels are made from food crops like corn, some are made from byproducts like plant husks, but CSHL Professor Rob Martienssen is using something unique: duckweed.
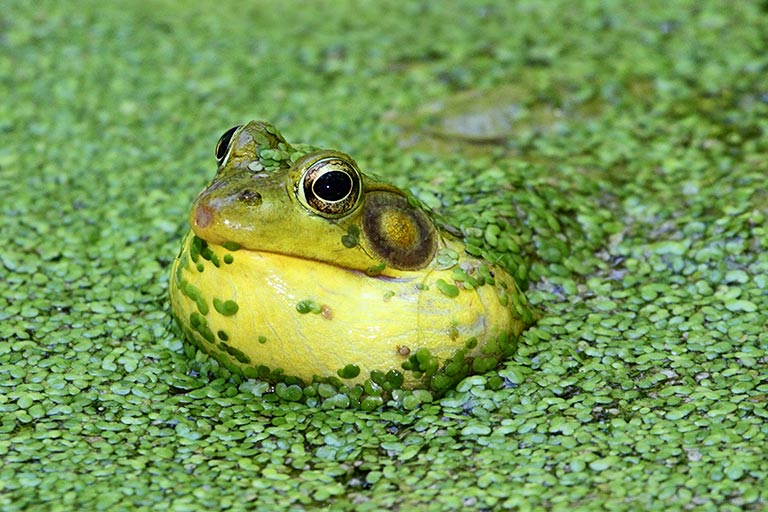
Duckweed has a lot more pros than cons as a biofuel producer. It reproduces clonally, making copies of itself so quickly that it doubles its mass every two days. One drawback with duckweed, however, is that it uses a lot of carbon dioxide, which it takes up to make starch–and starch requires lots of energy to turn into fuel. Martienssen and his team are using the power of genetic information to side-step this problem altogether and make duckweed an even more promising biofuel crop. Learn how, and more about his big plans for this tiny plant in this new episode of Base Pairs.
BS: Hey everyone, I’m Brian.
AA: And I’m Andrea.
BS: And today, we’re going to talk about… well… let’s just cut straight to the clips.
Newsreel supercut: “Co2” “Greenhouse gasses” “Record levels” “Rising temperatures” “Global warming.”
BS: All these soundbites, as you can probably guess, feature folks who are very worried about record-climbing levels of greenhouse gases, which scientific consensus says is tied to warmer, worrying shifts in our planet’s climate.
AA: Now, I’ll cut in to say that Base Pairs is not “the podcast about climate change” – this is the podcast about the power of genetic information, and we have a story for you about how diving into the genomes of plants could help in the fight against climate change. But first [pause] some stats:
BS: According to the US Environmental Protection Agency, global greenhouse gas emissions have spiked by about 90% since the 1970s, with emissions from fossil fuel combustion contributing about 78% of the that total increase. And if you’re wondering why burning these fossils fuels might shake up business-as-usual for our climate – put simply – we’re pulling billions of tons of carbon dioxide from out of the earth and releasing it into the air.
AA: The working theory is that high concentrations of greenhouse gases such as carbon dioxide can trap sun-delivered heat, and while a lot of that co2 gets reabsorbed by the processes of photosynthesis –
BS: Thanks, trees! Thanks, algae!
AA: – a lot also contributes to warming our oceans, which melts glaciers and permafrost, raising sea levels and causing some really weird weather.
BS: And that’s why shifting away from fossil fuels is a huge goal of the nations that signed the Paris climate accord.
AA: the Paris Agreement, as it is often called, was first signed by 196 international parties in 2015. Its central aim is keep the global temperature rise in this century well below 2 degrees Celsius — as measured from a baseline of pre-industrial levels.
BS: And according to experts around the world, abandoning fossil fuels in favor of biofuels will be instrumental in keeping that central aim a realistic possibility. The idea is that biofuels, which come from carbon-consuming plants, can be “carbon neutral” – that is to say, the amount of carbon dioxide released by burning them is mitigated by how much those plants absorb before they’re burned. Compare that to burning fossil fuels, which simply releases co2 that was trapped in the earth for millions of years… and you can see why the former would be preferable.
OD: The basic aspect is that biofuels replace fossil fuels. Being renewable, [biofuels] come from plants, they go back to plants or to residues that go back into the soil and so on at some stage, so basically it’s a virtuous cycle… Basically, bioenergy including biofuels are an essential component to achieve our climate change targets.
BS: That’s Olivier Dubois, of the United Nations’ Food and Agricultural organization… and I really hope I got his name right.
OD: some people would call me Olivier Dubious. I said no. I’m not dubious. Dubois. Dubois. Olivier Dubois. Not dubious. [laughter]
BS: (laughing) not dubious at all! In fact, Olivier is one of the FAOs leading experts on biofuel.
OD: I’m a Senior Natural Resources officer in FAO, and I currently coordinate the energy program including on bioenergy and biofuels in FAO… And… if we really want to reach the two degrees limit and even below two degrees from the Paris agreement, we need by 2030, twice as much bioenergy that we have now and four times more biofuels. And when you go to 2060, it’s four times more bioenergy and 10 times more biofuel.
AA: So, two times more bioenergy and four times as much biofuel?! But wait. Brian. It’s 2018. Is Olivier saying that the world is going to have to quadruple our biofuel production within the next decade? That’s a fairly tall order!
BS: It is! But Olivier told me that the UN currently estimates that biofuels only make up five to six percent of all fuel, so it’s not as tall of an order as you might think.
OD: Certainly less than 10% currently. Out of that, less than, much less than 5% are second generation. The bulk of the current biofuel production is basically first generation.
BS: So, apparently there are a LOT of different kinds of biofuel, and you can divide them into three distinct generations.
OD: within the biofuel sphere, you have biofuels produced out of let’s say food crops. You have those from starch like from corn or sugarcane, and then those that are produced from oil such as rapeseed, palm oil, soybeans. These are two major types of biofuels made out of food crops and they are called first generation biofuels.
Then you have another category which is the second-generation biofuels, which are usually considered to be those produced out of either residues from agriculture, food production, whatever biomass residues you have. It can also be from restaurants, reusable waste.
and then you have the more advanced which are like from algae and that kind of stuff, which is really currently in research.
AA: Wow! So, what’s holding us back? Why haven’t any of these biofuels already saved the planet, helping us break away from fossil fuels for good?
BS: Well that’s the thing. Each of these generations of biofuels have its drawbacks and limitations. The first generation – corn ethanol for instance – is easy for even undeveloped nations to pick up, but in doing so, they’re left with less cropland for growing foodcrops. Olivier told me that it’s actually rare for this to pose a problem, and really should be looked at on a community-by-community basis. But opponents of first generation biofuels will highlight this food vs fuel dilemma.
Likewise, with second generation biofuels –
AA: Those are the fuels made from food industry residues… so hay bales, corn husks, or used cooking oil?
BS: And from grasses and trees, but yes, food industry residues are the source of a lot of second generation biofuels. For them, the potential problems double:
OD: concerning the second generation biofuel which people say, “Well, it’s fantastic. it doesn’t have conflict with food.” Well, no direct, but indirectly you may have conflict because the residues from crops are often used for soil management. It’s the cheapest fertilizer for small scale farmers in developing countries. The straw can protect the soil from rain, for example, and you can also use it a lot as animal feed. From FAO’s work, we know that in developing countries, 30 to 40 percent of the animal feed for small scale herders in developing countries come from the residues from the farm.
BS: So even in this case, you run into the food vs fuel dilemma. The second problem is that lignin – that’s the tough, stringy parts like stalks, husks, and grasses – is SUPER difficult to break down. Doing this sustainably takes a lot of technological investment, limiting cellulosic fuel development to the wealthiest of nations.
AA: Ok but what about the third generation? I heard Olivier mention algae and that sounds promising! I imagine that you can farm algae in places where foodcrops can’t grow, so that at least gets rid of one problem.
BS: You’re right. But then you’re still left with high costs of a different kind:
OD: Normally these advanced biofuels, they require a lot of energy. The algae biofuel requires so much energy that if you do the balance you may use more energy than you produce. the thing is bioenergy… is a very complex and multifaceted topic.
BS: So, those third generation biofuels, while promising, currently require a lot of energy to produce in the first place.
AA: That’s exactly the problem that CSHL Professor Rob Martienssen is working on. His team is searching for a way to make a more efficient, sustainable advanced biofuel not from algae, but from another aquatic plant. And it’s no coincidence that they’re both aquatic—plants that live in or on water have unique features that are useful for making biofuels.
RM: Aquatic plants like to absorb a lot of CO2. Because they live on water, they don’t need to worry about water loss through transpiration [AA: that’s plant sweat, essentially] through their stomata [AA: the pores through which plants both sweat and breathe] and so they can keep their stomata, their guard cells, open all the time and so they can suck in huge amounts of CO2.
BS: Cool! So, what’s this other aquatic plant Rob’s interested in?
AA: Well, when he was telling me about the advantages of aquatic plants for sucking carbon out of the atmosphere, he was actually talking about a plant that lived tens of millions of years ago.
BS: Oh… then how is that going to help us make better biofuels now?
AA: The plant Rob’s team is studying in his lab is has features that are very similar to those of this ancient species of… pond scum, basically, called Azolla. It was a tiny fern that grew on the surface of freshwater, and it seems to have spurred an enormous change in climate. This plant’s impact is thought to have been so dramatic that this climate shift became known as the Azolla Event.
RM: About 50 million years ago roughly, a bit less, during the Eocene, the level of CO2 in the earth’s atmosphere was much, much higher than it is now. — It was about 3,600 ppm.
BS: 3,600 part per million!! Right now, we’re worrying that the level of atmospheric carbon recently exceeded 400ppm.
AA: As you might guess, the climate was way warmer than it is today because of all of that heat-trapping carbon in the atmosphere.
RM: The surface temperature of the Arctic Ocean was something like 13 Celsius, which is extremely high. There were hippopotamuses and palm trees in the Arctic and it was a hot house climate. But the Arctic Ocean was actually surrounded by land at the time, and so it was somewhat fresh water and so could support the growth of these freshwater aquatic plants, which are extremely rapidly growing.
BS: While hippos in the Arctic is one of the more fun effects of warming climates that I’ve heard about, we still need to avoid atmospheric carbon levels getting anywhere near that high again right now. But how do we know that Azolla, this ancient pond scum, should get so much of the credit for reducing the amount of carbon in the atmosphere?
AA: When plants suck carbon out of the atmosphere in the form of CO2, the carbon doesn’t disappear—it gets converted into plant matter. So, all of that CO2 that was in the atmosphere millions of years ago got converted to tons upon tons of Azolla. And a lot of it is still up there in the Arctic.
RM: Geologists went to look at the fossil record by drilling down through the Arctic sea bed and from cores that they dug up there they found, largely from pollen samples, that there were mats of Azolla 8 to 20 meters thick covering the Arctic Ocean for many hundreds of thousands of years. And this was enough to absorb a huge amount of CO2 and actually reduce the level and the temperature of the earth to more or less what it is now. — In a sense, aquatic plants have this capability of changing the climate.
BS: That’s amazing!
AA: It is! But, there’s a big catch.
RM: The time that we have to do this is not as long as was available in the Eocene when it took more than a half a million years to complete this. So, we need obviously to do something with a bit more of an engineered strategy to be able to use aquatic plants in this way, but it is an exciting prospect.
AA: Rob is using a type of aquatic plant very similar to Azolla for his research. It’s called duckweed. Rob’s team has been diving deep into the genomes of various duckweeds to find ways to make them an even better tool for sucking carbon out of the atmosphere and turning it into fuel. Duckweeds are really common—I’m sure you’ve seen them.
RM: Ponds on golf courses are classic examples because of all the fertilizer that’s put on the grass runs off into the pond. — Duckweeds, or the Lemnaceae, are the smallest flowering plants, but also the fastest growing. — Now, although they weren’t actual Lemnaceae, these — Azolla — were aquatic ferns — they grew very similarly as far as we can tell.
BS: I’ve definitely seen duckweed. It is a very modest-looking plant, but being the fastest-growing plant in the world is quite a feat. How do they do it?
AA: They clone themselves.
BS: Really?
AA: Really. For both duckweed and Azolla…
RM: …the clonal reproduction aspect of it is why they grow so fast.
AA: By cloning itself, duckweed is able to double in biomass every two days!
BS: Cloning itself—so this is different from the highly-engineered way that scientists created Dolly the famous cloned sheep, for example. Some plants can naturally sprout clones of themselves. Similarly, some of you may have used cuttings of houseplants to grow new plants. That’s a form of cloning, too.
AA: Duckweed is a plant that clones itself naturally. While the Azolla Event helped inspire Rob to look into duckweed as a potential biofuel, it was clonal reproduction that really got him interested in duckweed. He’s kind of a plant clone enthusiast, and after talking with him, I can see why. Clones offer some huge advantages.
RM: My lab really works on different types of plant reproduction and the genetic and epigenetic mechanisms that underlie it. We actually always had an interest in plants growing from clones and the epigenetic mechanism they have.
AA: When you look at the net amount of energy that various biofuel crops produce, you see that the ones that come out on top are major food crops, which run into the food vs. fuel issues you talked about with Olivier. But they have something else in common, too: the best energy crops are clones.
RM: Right now, the most successful biofuel feedstocks are sugarcane and oil palm—both of which are clonal, by the way, and both of which can produce between five and 10 times as much energy as what you put in.
BS: Those are some impressive numbers! Clonal reproduction makes that big of a difference?
AA: It can. While it’s not the only factor that makes oil palm and sugarcane good biofuel crops, cloning can greatly improve a plant’s yield. One reason for that is when you find one particularly high-yielding plant, you can then make genetically identical copies.
BS: It’s kind of like if the Yankees were able to clone Babe Ruth, except instead of racking up home runs, you get more palm oil or sugar or whatever the crop is. Duckweed has a big advantage, then, as a clonally reproducing plant that isn’t a big food crop. How does duckweed stack up in terms of net energy production?
AA: It’s hard to say exactly, because Rob’s team is still working on making duckweed a better biofuel crop. For example, when duckweed takes up CO2, it mostly uses that carbon to make starch, which requires a lot of processing to turn into fuel. But Rob and his team thought, is there a way that we could get duckweed to make oil instead?
BS: Oil is a much better starting material for biofuel than starch, so that would be a major improvement. But that’s also a pretty big change to make to a plant. How is he going to do that?
AA: One particularly appealing way is to create a whole new chromosome that contains the instructions for making oil using CO2. But you can’t just stick a whole new chromosome into any plant and expect it to work out—unless it reproduces clonally.
RM: We’re very interested, for example, in using artificial chromosome technology where you can literally build your own chromosome. — Another advantage of clonal growth is that it can tolerate additional chromosomes.
BS: It’s not surprising that adding an entire chromosome could cause problems, but why are clones better at dealing with those problems?
AA: It has to do with how sexual reproduction works. Both parents contribute one copy of each their different chromosomes.
BS: Chromosomes are big packages of DNA, basically.
AA: Yeah, and each chromosome from the mother’s egg pairs up with the corresponding chromosome from the father’s sperm to create a combination of both parents’ DNA in the offspring. But, if you toss in an extra chromosome, the whole pairing process gets thrown off.
BS: Ah, so if the plant reproduces clonally instead of sexually, it can just skip that part and make copies of all of its chromosomes, including the extra chromosome.
AA: Right. Rob’s team is still working toward this additional chromosome approach, but in the meantime, they have successfully gotten duckweed to produce oil using a different technique. There’s this gene in corn called WRINKLED1 that’s known to be involved in a biological pathway that produces oil.
RM: Sure enough, if you express WRINKLED1 in duckweed, you do get oil. You don’t get a huge amount of oil and part of the reason for that is that you slow down growth a great deal and this is a predicted consequence of expressing only part of the oil pathway, not the whole thing.
BS: This approach is kind of like taking a shortcut, it seems. Rob wanted to see if duckweed could produce oil, which is a process that involves a number of genes, but this WRINKLED1 gene is the key player. Putting WRINKLED1 into duckweed was enough to get it to make oil, but shortcuts often come with a cost. In this case, the cost was slower growth.
AA: Yeah, this approach was a good kind of proof-of-concept that it is possible to get duckweed to make oil. But as you discussed with Olivier, efficiency is the big problem that third generation biofuel crops like algae and duckweed face.
BS: It’s important to get more energy out of these plants than we put into growing them, and slower growth gets in the way of that.
AA: This is where the extra chromosome approach is useful. Instead of moving just one gene from this oil-producing pathway into duckweed, building a whole chromosome would allow Rob and his team to move all of those supporting genes in the pathway along with it.
RM: We think we can build our own chromosomes in duckweed, which would be very convenient for being able to move entire biosynthetic pathways from one organism to another.
BS: In other words, Rob wants to give duckweed the genetic tools it needs to be better at producing oil.
AA: Right.
AA: There is another angle on this duckweed oil production issue, though.
RM: [at lecture] It turns out that if you grow them under the right conditions, you can overcome much of that growth deficit. One of those conditions, excitingly for us, is growing them in high CO2.
BS: That is very convenient, since we’re living in relatively high CO2 conditions right now.
AA: Relatively…. but to improve duckweed’s growth rate, Rob needs a way to create even higher CO2 conditions. You might have noticed that he sounded a bit different in that clip, and that’s because it’s from a public lecture that he recently gave here with Frank O’Keefe, who’s the CEO of a company called Infinitree that found a way to do just that. Here’s Frank:
FO: What we discovered was remarkable and gave us hope that we could feed greenhouses, vertical farms, with cheap, low-energy CO2.
AA: Frank and his colleagues at Infinitree developed this absorbent material that works as a “humidity swing,” that’s what they call it. When the material is dry, it captures CO2, and when it’s wet, it releases that CO2.
BS: Wow, that seems like it would work well for releasing CO2 into a greenhouse, where it tends to get really humid anyway.
AA: Once Rob and Frank started talking with each other, it became obvious that their projects fit together quite nicely.
RM: We hadn’t really been thinking about a CO2 system like that, but it really makes a huge amount of sense because now that technology allows essentially passive capture of CO2 to make the CO2 concentration much higher and we’ve done some experiments. It makes the duckweed grow much faster, it increases oil in our special strains.
BS: Since this material essentially captures CO2 passively—meaning without putting in more energy—that could really help make duckweed a more sustainable biofuel.
AA: Frank also pointed out the advantages of duckweed’s very small stature. You can grow it in shallow, stackable containers using the system that his team designed.
FO: The reason we would use that plant is that it so voraciously consumes CO2, that for us to grow it in layers—25 layers, per our design, so you’ve got a vertical farm of 25 growth layers—would enable us to put away a lot of CO2.
BS: These are some pretty big plans for the world’s tiniest flowering plant. And I feel good about it! But is it going to be ready for that 2030 goal that I discussed with Olivier at the top of the show?
AA: Even Rob believes that duckweed is not “the one fuel to rule them all” so to speak. It will have to be part of a larger effort that includes other types of biofuels as well.
RM: It’s not like we’re gonna be growing huge amounts of duckweed next week worldwide, but we certainly think of it as a big new potential component. Biofuels, the oil companies are facing a requirement to use a pretty high percentage of biofuels in the next few years, just legislatively they have to do this. So they’re very interested in all sorts of different ways of producing a large amount of biofuels.
BS: That’s great to hear. As Olivier was telling me, the world needs to focus on adopting any kind of biofuel first, before research that improves the efficiency of newer biofuels can start to play its role on a global scale.
OD: Basically, there’s no one size fits all and there’s no “either or” … You can have unsustainable and non-sustainable, and sustainable biofuels in whatever category. But… you need all types of biofuels to really meet that [2030] target. I mean, you will never reach a sufficient level of second generation in 10 years time to really make up for the shortfall if you forget about the first generation. Right? What really matters is… the understanding of the situation for each country level… or even in terms of environment…so that these biofuels are sustainable.
AA: Absolutely. Duckweed is part of this third generation of advanced biofuels, which come with many advantages and are still being improved upon. But if we’re going to have a shot at making that 2030 goal, we’ll need those earlier generations of biofuels in the meantime.
BS: I hear ya. For me, this duckweed project of Rob’s represents that future of “clean” and carbon-neutral fuel we’re looking forward to. But we’re not there yet. We’re going to have to get there in steps, and research makes sure we always have cleaner more energy-efficient steps waiting just ahead of us.
Written by: Sara Roncero-Menendez, Media Strategist | publicaffairs@cshl.edu | 516-367-8455