Nobel laureate Richard Roberts explains the importance of adenovirus in the landmark discovery of RNA splicing and his love for ‘failed’ experiments.
Adenoviruses are best known for their role in causing illness. They may have been to blame for some of the colds and sore throats you’ve suffered through. But around CSHL, the adenovirus is far from a villain—it’s a hero so celebrated that its likeness adorns a beloved spot on campus (it’s the hexagonal object at the very top of the rod in the photo above).It was through experiments on adenovirus that scientists discovered the phenomenon now known as RNA splicing, a key to the genome’s ability to generate astounding complexity. Except at first, these experiments appeared to have been utter failures, explains Richard Roberts, who shared the 1993 Nobel Prize in Physiology or Medicine with Philip Sharp of MIT for this immense discovery.
One day in 1977, Roberts says, a postdoc named Rich Gelinas working in his lab at CSHL came to him completely puzzled by the results of an experiment on adenovirus. Where he expected to see 15 or 20 spots in his test results, he saw just one. Roberts remembers that he “immediately said, ‘Better go back and do it again because obviously something went wrong along the way.’” The repeated experiment, even more curiously, yielded precisely the same result.
Each spot in this kind of test represents a segment of an individual RNA, a molecule that helps ferry genes’ instructions from DNA to the machinery that builds proteins, the workhorses of the cell. Roberts and his team were trying to understand the basic process of how information encoded in DNA is translated into proteins. Adenovirus was such a useful model organism because unlike many other viruses and bacteria, it has linear DNA, just like humans do. Yet it’s a simple entity that makes just a few dozen proteins—perfect for exploring this basic process.
Why CSHL put an adenovirus model on top of a gazebo
Adenovirus actually got its start at CSHL as a system for studying cancer. Along with a monkey tumor virus called SV40, it was used extensively in early “Cancer War” research and led to a number of important discoveries in the cancer field. It was these discoveries that spurred the creation of the adenovirus model that still adorns the gazebo on top of CSHL’s Water Treatment Plant. Elizabeth Watson wrote in her book Houses for Science: A Pictorial History of Cold Spring Harbor Laboratory that, “When the little copper model of an adenovirus was placed on top of the gazebo in 1976 no one at the Laboratory would have predicted that the ongoing experiments with this virus would very soon revolutionize thinking about gene structure.”
At the end of this experiment, Roberts and Gelinas expected to see many spots because they knew that adenovirus makes dozens of proteins. And they knew that there are different RNAs that carry the instructions for each different protein. This result showing just one kind of RNA didn’t make sense. According to Roberts, this usually means one of two things happened with your experiment: either you “screwed it up” or “nature is trying to tell you something.”
This time, it was the latter—nature was telling them about the strange, ingenious, and never-before-recognized process of RNA splicing. It turns out that one gene doesn’t necessarily contain the instructions for only one protein, as was previously thought. Pieces of information in a single gene can be arranged, or spliced, in different combinations to produce a variety of proteins.
This rearranging of a gene’s information can’t happen in DNA, since that’s the permanent copy. Instead, splicing happens after the gene’s information gets copied into an RNA molecule. These raw RNA molecules then get chopped up into modular bits of information and then spliced together into unique combinations. That one spot that Roberts and his postdoc saw? It was an RNA “module” that is part of multiple RNAs—a clue that the final, “edited” protein-encoding RNA messages of genes are formed through splicing.
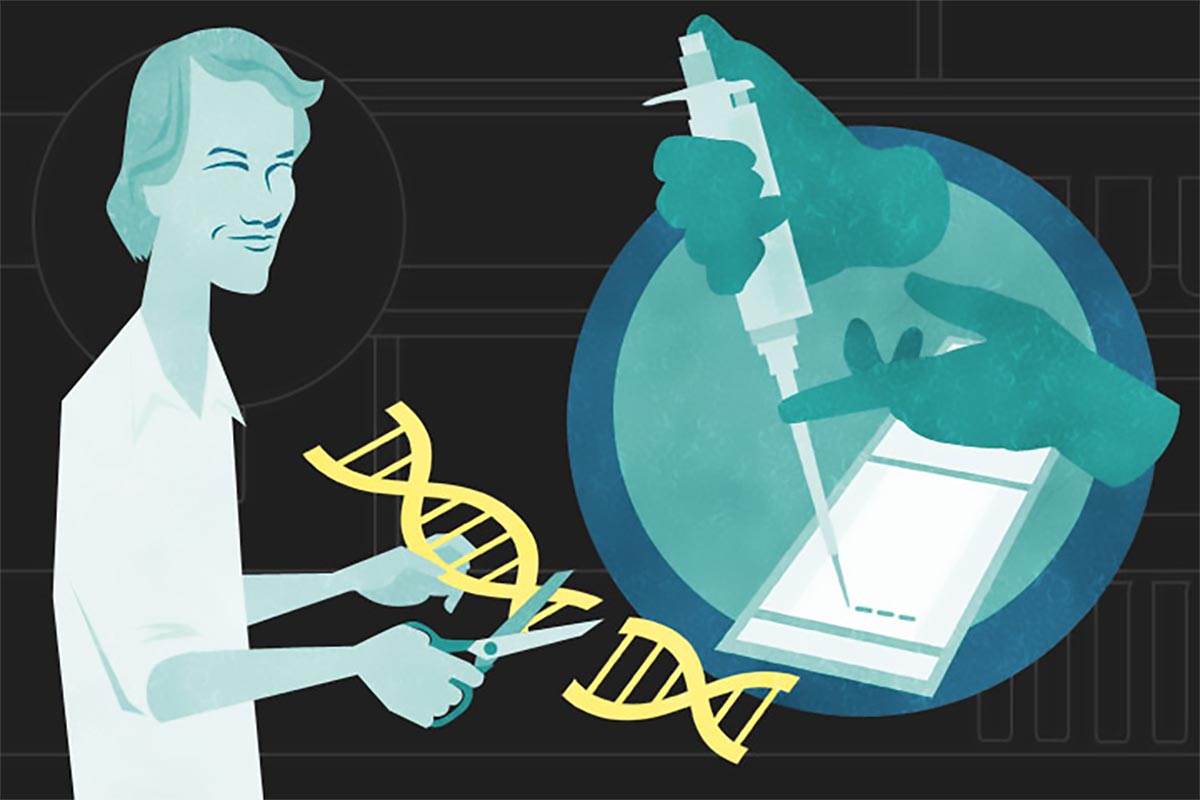
“This is why I love failure when you’re doing experiments,” Roberts says. Nature was indeed trying to tell them something. They had stumbled upon one of the reasons that the number of genes that an organism has doesn’t always correspond to the complexity of that organism. Humans have about 10,000 fewer genes than corn, for example, and this is one of the reasons. Our 21,000 genes contain information for the manufacture of many, many times more proteins—the number is still unknown, but is estimated at anywhere from 250,000 to one million!
Just as important, errors in splicing can cause disease. The leading genetic cause of death among infants is a disease called spinal muscular atrophy, and central in its pathology is an error in RNA splicing. Knowledge of splicing has made it possible to develop a drug called nusinersen, which corrects the error and is now in late-stage clinical trials—and achieving amazing results so far, in very sick infants and young people. There are many players in that story who deserve recognition for their role in making the drug possible, a story we will be writing more about in the months to come. Certainly, adenovirus is one of those players—perhaps the most overlooked.